Editor-in-Chief : V.K. Rastogi
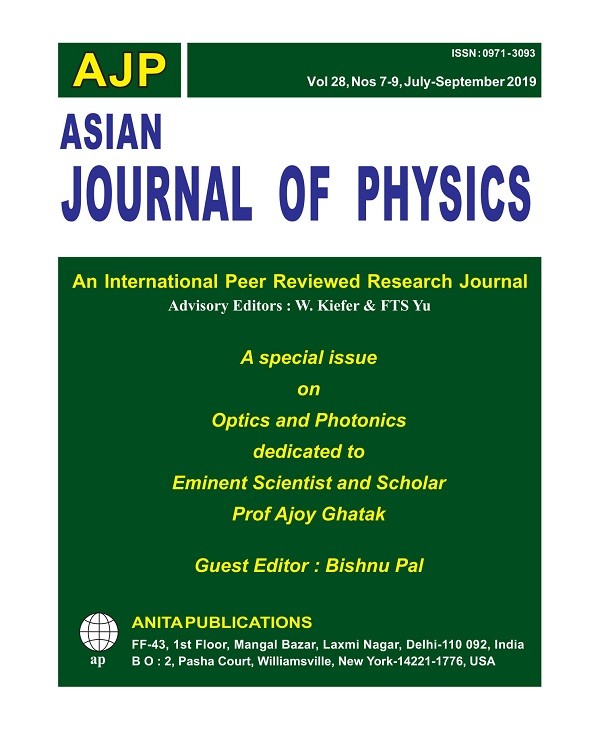
ASIAN JOURNAL OF PHYSICS
An International Peer Reviewed Research Journal
Frequency : Monthly,
ISSN : 0971 – 3093
Editor-In-Chief (Hon.) :
Dr. V.K. Rastogi
e-mail:[email protected]
[email protected]
AJP | ISSN : 0971 – 3093 Vol 28, Nos 7-9, July-September, 2019 |
Asian
Journal of Physics
A special issue on
Optics and Photonics
dedicated to
Eminent Scientist and Scholar Prof Ajoy Ghatak
M N Saha Distinguished Professor of NASI (The National Academy of Sciences, India)
and Formerly Professor of Physics at IIT Delhi
on his 80th birthday Nov 9, 2019
Anita Publications
FF-43, 1st Floor, Mangal Bazar, Laxmi Nagar, Delhi-110 092, India
Asian Journal of Physics
(A Publication Not for Profit)
Volume 28, Nos 7-9 (2019)
CONTENTS
Editorial
Guest Editorial
About the Guest Editor
About Prof Ajoy Ghatak
Ajoy K Ghatak – a physicist and teacher par excellence
Pankaj Kumar Choudhury
471
O-band Bi-doped fibre amplifiers: Present and Future
N K Thipparapu, J K Sahu and David N Payne
475
Light propagation through graded-index fibers: Impact of self-imaging on the nonlinear phenomena
Govind P Agrawal
487
A world without fibre Bragg gratings
Raman Kashyap
499
Twisted light: beams carrying orbital angular momentum
Siddharth Ramachandran
509
High-efficiency ring similariton fiber laser with nonlinear spectral compression
A S Abramov, D A Korobko, I O Zolotovskii and A A Sysoliatin
517
Blending random lasers and second order nonlinearities in neodymium-doped crystalline powders
Anderson S L Gomes, Lauro J Q Maia, André L Mouraand Cid B de Araújo
529
Towards stable delivery of high energy through background-guided similariton pulses
Piyali Biswas and Somnath Ghos
541
Fibre optic sensors – an exemplar on the routes to applications
Brian Culshaw
555
Fibre optic chemical sensors for environmental monitoring using sensitive nano-material coatings
Kasun Prabuddha Dissanayake, Papiya Dhara, Rahul Kumar, Souvik Ghosh, Hien T Nguyen, Tong Sun, and Kenneth T V Grattan
567
Tapered thin core fiber based highly sensitive twist sensor without temperature Crosstalk
Wenjun Ni, Baocheng Li, Chenlu Wang, Yu Zheng, Ran Xia,Yiyang Luo, Chunyong Yang, Perry Ping Shum
579
Cavity ring-down sensing techniques – A review
S O Silva, M B Marques, J L Santos, P A S Jorge and O Frazão
589
Manipulation of vector beams produced in optical fiber and an application as strain sensor
Partha Roy Chaudhuri, Saba N Khan and Sudip K Chatterjee
605
Optical Tweezers – the invention of which got the 2018 Nobel Prize in Physics
Pradeep Kumar Gupta
623
Low-power and high-frequency optogenetic control of spikingcin ultrafast red-shifted Chrimson-expressing neurons
Himanshu Bansal, Neha Gupta and Sukhdev Roy
631
All-dielectric metasurface for complex-amplitude modulation for linearly polarized light
Jangwoon Sung, Gun-Yeal Lee, Jongwoo Hong, Hyunwoo Son, and Byoungho Lee
645
Metasurfaces for magnetic field enhancement
Keshav Samrat Modi, Jasleen Kaur, Satya Pratap Singh, Umesh Tiwari and Ravindra Kumar Sinha
655
Fano Resonances in Terahertz Metamaterials
Subhajit Karmakar, Ravendra K Varshney, Dibakar Roy Chowdhury and Bishnu P Pal
663
Wavefront aberrations with incomplete Hartmann-Shack sensor data
Abbas Ommani, Natalie Hutchings, Ritambhar Burman, Damber Thapa and Vasudevan Lakshminarayanan
689
Review of optical image encryption schemes based on fractional Hartley transform
Phool Singh, A K Yadav, Sunanda Vashisth and Kehar Singh
701
Axial vs radial junction nanowire solar cell
Vidur Raj, Hark Hoe Tan and Chennupati Jagadish
719
Design, characterisation and optimisation of silicon-on-insulator (SOI) based photonic devices
Weifeng Jiang, Souvik Ghosh and B M A Rahman
749
Are optical quantum information processing experiments possible without beamsplitter?
Kishore Thapliyal and Anirban Pathak
761
About the Guest Editor
Bishnu Pal born on Dec 3, 1948 in Shillong India is currently Professor of Physics and Dean of Academics at Mahindra École Centrale Hyderabad, India. He has been Former Chair of Physics Department (2007-2011) and Computer Services Centre at IIT Delhi (2003-2006) India, Member Board of Directors of OSA The Optical Society (Washington, USA, 2009-2011), Fellow of OSA the Optical Society (USA) and SPIE The International Society for Optics and Photonics (USA), Distinguished Honorary Member of Royal Norwegian Academy of Sciences and Letters (DKNVS, Norway), Senior Member of IEEE (USA) and Distinguished Lecturer of IEEE Photonics Society (2005-2006), Fellow and President of Optical Society of India (2012-2015), Alexander von Humboldt Fellow (1982-83, Germany) and Fulbright Scholar at NIST Boulder CO (1991, USA), PDF and latter Visiting Professor of Royal Norwegian
CSIR (NTNF, Oslo, 1975-77) at Norwegian University of Science and Technology Trondheim Norway, awardee of Esther Hoffman Beller Medal of OSA, The Optical Society (Centenary Year 2016, USA), Invited faculty at the ICO Winter School in Optics held at ICTP 1998, Awardee of Homi Bhabha award in Applied Physics of UGC (India, 2006), Om Prakash Bhasin award in Electronics and Information Technology (India, 2013), Khosla Research Award of IIT Roorkee (India, 2014) for lifetime contributions in research, Prof Y T Thathachari award for excellence in Physical Sciences (India, 2010) of Brhamara Trust (Mysore), CEOT award of IETE (India, 2010) for his contributions to Optoelectronics Devices, Co-recipient of the Fiber Optics Person of the Year award (1997) established by Lucent Technology in India and Optics award of Optical Society of India (OSI, 2010) for lifetime achievements.
Prof Ajoy Ghatak
Professor Ajoy Ghatak is an eminent Indian scientist and a teacher per excellence on Optics and Photonics and celebrated former Professor of Physics at IIT Delhi, who is known all over the world for his significant research work on photonics, fiber optics and education in India and for his lucidly written outstanding books on Optics, Lasers, Quantum Mechanics and Relativity. Professor Ghatak has created a legacy of pride for the country and is commended as “one of the most eminent scientists in Optics and Photonics in general of all times in India”. Professor Ghatak was born on November 9, 1939 at Lucknow. He received his B Sc from Agra College, M Sc from Delhi University and Ph D from Cornell University, USA.
After a short stint as a Research Associate at the Brookhaven National Laboratory, USA, Professor Ghatak joined the Indian Institute of Technology Delhi, in 1966, where he was a Professor of Physics from 1974 till his retirement. Professor Ghatak has more than 170 research papers to his credit in various International journals and more than 20 books.
His book on Optics (translated into Chinese and Russian also) is very extensively used all over as a text in undergraduate teaching and in addition one on Fiber Optics, and another on Optical Electronics, and one on Quantum Mechanics are also very widely used for senior UG and PG courses as texts in these subjects. His research contributions have been recognized through his induction as a Fellow of OSA.The Optical society, SPIE, NASI, OSI, and numerous international and national prestigious awards like SSB award of CSIR, Galileo Galilei award of ICO, Beller medal of OSA, SPIE Educator award and MN Saha award of UGC. As a current M N Saha Chair Professor of NASI he continues to contribute tirelessly and significantly to the progress and dissemination of knowledge in Optics, Photonics, and Quantum Physics. Even at his late-70 age, he can be seen frequently travelling to various colleges, institutions far-and-wide across the country to deliver tutorial level talks and also to disseminate knowledge on contributions made by Indian Scientists to motivate generation X students to take up teaching and research as a profession. He has published several popular science level books on Albert Einstein based on his personal research on Einstein’s monumental and iconic research. One of Prof Ghatak’s seminal contributions in Guided Wave Optics has been his analysis and work on the physics of leaky modes in optical waveguides in a single-authored paper that had appeared in the Springer journal: Optics and Quantum Electronics in its September 1985 issue. This work eventually led to development of a Matrix method (IEEE J. Lightwave Tech. May 1987) for treating optical waveguides (and also optical fibers), which became an extremely powerful research tool for designing a variety of guided wave components and specialty optical fibers. This method found extensive use by other researchers including Prof Ghatak himself in numerous international journal publications on optical waveguide and fiber designs for realization of components thereof including quantum well devices.
Asian Journal of Physics |
Vol. 28 Nos 7-9 (2019) 471-474 |
Ajoy K Ghatak – a physicist and teacher par excellence
Pankaj Kumar Choudhury
Recalling my acquaintance with this highly acclaimed gentleman, Ajoy K. Ghatak. My memory goes back to the days of Masters’ studentship in the year 1986 while coming across the subject, Quantum Mechanics. To some extent, the subject was interesting to me, and we had to study this in all four semesters in a two-year M. Sc. course. On the advice by our course lecturer, we had to go through certain sections, particularly the Schrödinger equation and its use in treating some of the problems in Quantum Mechanics, of the book by Ghatak & Loknathan – a very popular duo name who not only did write on Quantum Mechanics, their authorities can be witnessed in some other subject arena (of physics) as well through multiples of published volumes. That was my first interaction with Ghatak Sir, though not face-to-face. Through even books it left like a very prominent impression of his being an academic giant. During that time, several books were in record realised by leading publishing firms, and authored by this duo.
Ajoy K Ghatak – a physicist and teacher par excellence.pdf
Pankaj Kumar Choudhury
Asian Journal of Physics |
Vol. 28 Nos 7-9 (2019) 475-484 |
O-band Bi-doped fibre amplifiers: Present and Future
N K Thipparapu, J K Sahu and David N Payne
Optoelectronics Research Centre,
University of Southampton, Highfield, Southampton, SO17 1BJ, U.K.
Invited Article dedicated to Prof Ajoy Ghatak on his 80th Birthday
This paper reviews and discusses recent developments in O-band Bi-doped fibre amplifiers. We present the performance of Bi-doped fibre amplifier in optical fibre communication systems and provide future prospects. © Anita Publications. All rights reserved.
Keywords: Fibre optics amplifiers, Fibre characterization, Fibre design and fabrication, Fibre materials
References
1. Mears R J, Reekie L, Jauncey I M, Payne D N, Low-noise erbium-doped fibre amplifier operating at 1.54 μm, Electron Lett, 23(1987)1026-1028.
2. Agrawal G P, Optical Communication: Its History and Recent Progress, in Optics in Our Time, (Cham: Springer International Publishing), 2016, pp 177-199.
3. Cisco Visual Networking Index: Forecast and Trends, 2017–2022 White Paper, https://www.cisco.com/c/en/us/solutions/collateral/service-provider/visual- networking-index-vni/white-paper-c11-741490.pdf
4. Li G, Bai N, Zhao N, Xia C,Space-division multiplexing: the next frontier in optical communication, Adv Opt Photon, 6(2014)413-487.
5. Ellis A D, Suibhne N Mac, Saad D, Payne D N, Communication networks beyond the capacity crunch, Philos Trans R Soc, A 374(2016)20150191; doi.org/10.1098/rsta.2015.0191
6. Richardson D J, New optical fibres for high-capacity optical communications, Philos Trans R Soc, A 374(2016) 20140441; doi.org/10.1098/rsta.2014.0441 .
7. Winzer P J, Neilson D T, Chraplyvy A R, Fiber-optic transmission and networking: the previous 20 and the next 20 years [Invited], Opt Express, 26(2018)24190-24239.
8. Daniel Daems, Increasing data traffic requires full spectral window usage in optical single-mode fiber cables, commscope, 2019 https://www.fibre- systems.com/sites/default/files/content/white-paper/pdfs/Spectral_Window_Usage_In_SM_Fiber_Cables_WP-110966-EN%20%285%29_0.pdf
9. Turkiewicz J P, Opportunities for the out of the 1550 nm Window transmission, APGOŚ, 2019, pp 4-7; DOI: 10.5604/01.3001.0013.2538
10. Desurvire Emmanuel, Erbium Doped Fiber Amplifiers- Principles and Application, Hoboken, NJ: John Wiley & Sons, Inc. ISBN 0-471-58977-2,2002.
11. Thipparapu N K, Development of Bi-doped fibre amplifiers and lasers & broadband Er-doped multi-element fibre amplifiers (Doctoral dissertation, University of Southampton), 2018.
12. Becker P M, Olsson A A, Simpson J R, Erbium-doped Fiber Amplifiers: Fundamentals and Technology (Academic, Press), 1999.
13. Elad Mentovich (Mellanox) & Chris Cole (Finisar), “1300nm vs. 1550nm,” 5th International Symposium for Optical Interconnect in Data Centres, Meeting Room J2, ECOC Exhibition 2017, https://www.finisar.com/sites/default/files/resources/1310nm_vs_1550nm_ecoc2017.pdf
14. Banerjee A, Park Y, Clarke F, Song H, Yang S, Kramer G, Kim K, Mukherjee B, Wavelength-division-multiplexed passive optical network (WDM-PON) technologies for broadband access: A review [Invited], J Opt Netw, 4 (2005)737-758.
15. Payne D N, Gambling W A, Zero material dispersion in optical fibres, Electron Lett, 11(1975)176-178.
16. Niazi S A, Zhang X, Xi L, Munir A, Idrees M, Khan Y, A viable passive optical network design for ultrahigh definition TV distribution, Advances in Optoelectronics, 2013, Article ID 219271, 6 pages; doi.org/10.1155/2013/219271
17. Tanaka K, Agata A, Horiuchi Y, IEEE 802.3av 10G-EPON standardization and its research and development status, J Lightwave Technol, 28(2010)651-661.
18. Thipparapu N K, Jain S, Umnikov A A, Barua P, Sahu J K, 1120 nm diode-pumped Bi-doped fiber amplifier, Opt Lett, 40(2015)2441-2444.
19. Bufetov I A, Melkumov M A, Firstov S V, Riumkin K E, Shubin A V, Khopin V F, Guryanov N, Dianov E M, Bi-doped optical fibers and fiber lasers, IEEE J Sel Top Quantum Electron, 20(2014)111-125.
20. Dianov E M, Bismuth-doped optical fibers: a challenging active medium for near-IR lasers and optical amplifiers, Light Sci Appl, 1(2012) e12 ; doi.org/10.1038/lsa.2012.12
21. Thipparapu N K, Guo C, Umnikov A A, Barua P, Taranta A, Sahu J K, Bismuth-doped all-fiber mode-locked laser operating at 1340 nm, Opt Lett, 42(2017)5102-5105.
22. Thipparapu N K, Wang Y, Wang S, Umnikov A A, Barua P, Sahu J K, Bi-doped fiber amplifiers and lasers [Invited], Opt Mater Express, 9(2019)2446-2465.
23. Firstov S, Alyshev S, Melkumov M, Riumkin K, Shubin A, Dianov E, Bismuth-doped optical fibers and fiber lasers for a spectral region of 1600–1800nm, Opt Lett, 39 (2014)6927-6930.
24. Firstov S V, Alyshev S V, Riumkin K E, Melkumov M A, Medvedkov O I, Dianov E M, Watt-level, continuous-wave bismuth-doped all-fiber laser operating at 1.7 μm, Opt Lett, 40(2015) 4360-4363.
25. Kalita M P, Yoo S, Sahu J, Bismuth doped fiber laser and study of unsaturable loss and pump induced absorption in laser performance, Opt Express, 16(2008)21032-21038.
26. Yoo S, Kalita M P, Nilsson J, Sahu J K, Excited state absorption measurement in the 900-1250nm wavelength range for bismuth-doped silicate fibers, Opt Lett, 34(2009)530-532.
27. Riumkin K E, Melkumov M A, Varfolomeev I A, Shubin A V, Bufetov I A, Firstov S V, Khopin V F, Umnikov A A, Guryanov A N, Dianov E M, Excited- state absorption in various bismuth-doped fibers, Opt Lett, 39(2014) 2503-2506.
28 Dianov E M, Mel’kumov M A, Shubin A V, Firstov S V, Khopin V F, Gur’yanov A N, Bufetov I A, Bismuth-doped fibre amplifier for the range 1300-1340 nm, Quantum Electron, 39(2009)1099-1101.
29. Thipparapu N K, Umnikov A A, Barua P, Sahu J K, Bi-doped fiber amplifier with a flat gain of 25 dB operating in the wavelength band 1320–1360 nm, Opt Lett, 41(2016)1518-1521.
30. Thipparapu N K, Wang Y, Umnikov A A, Barua P, Richardson D J, Sahu J K, High gain Bi-doped all fiber amplifier for O-band DWDM optical fiber communication, in Optical Fiber Communication Conference (OFC) 2019, OSA Technical Digest (Optical Society of America, 2019), paper M1J.5.
31. Thipparapu N K, Wang Y, Umnikov A A, Barua P, Richardson D J, Sahu J K, 40 dB gain all fiber bismuth-doped amplifier operating in the O-band, Opt Lett, 44(2019)2248-2251.
32. Wang Y, Thipparapu N K, Umnikov A A, Barua P, Richardson D J, Sahu J K, Temperature Dependent Gain and Noise Figure Characteristics of O-Band Bismuth-Doped Fibre Amplifier, in European Conference on Optical Communications 2019, paper Th.1. C.2.
33. Taengnoi N, Bottrill K, Thipparapu N K, Umnikov A A, Sahu J K, Petropoulos P, Richardson D J, WDM Transmission with In-Line Amplification at 1.3μm Using a Bi-Doped Fiber Amplifier, J Lightwave Technol, 37 (2019)1826-1830.
34. Taengnoi N, Bottrill K, Hong Y, Thipparapu N K, Lacava C, Sahu J K, Richardson D J, Petropoulos P, Reach extension of PAM4 signals in O-band transmission by application of alternate-mark-inversion, in European Conference on Optical Communications 2019, paper P93.
35. Hong Y, Taengnoi N, Bottrill K R H, Sakr H, Bradley T D, Hayes J R, Jasion G T, Wang Y, Thipparapu N K, Umnikov A A, Sahu J K, Poletti F, Petropoulos P, Richardson D J, Dual O+C-band WDM transmission over 1-km hollow core NANF using an O-band Bismuth-doped fibre amplifier, in European Conference on Optical Communications 2019, paper Tu.2.E.1.
36. Fujimoto Y, Local Structure of the Infrared Bismuth Luminescent Center in Bismuth-Doped Silica Glass, J Am Ceram Soc, 93(2010)581-589.
37. Xu W, Peng M, Ma Z, Dong G, Qiu J, A new study on bismuth doped oxide glasses, Opt Express, 20(2012)15692–15702.
38. Sharonov M Y, Bykov A B, Petricevic V, Alfano R R, Spectroscopic study of optical centers formed in Bi-, Pb-, Sb-, Sn-, Te-, and In-doped germanate glasses, Opt Lett, 33(2008)2131-2133.
39. Dianov E M, Nature of Bi-related near IR active centers in glasses: state of the art and first reliable results, Laser Phys Lett, 12(2015)095106; doi.org/10.1088 /1612-2011/12/9/095106
40. Sokolov V O, Plotnichenko V G, Dianov E M, The origin of near-IR luminescence in bismuth-doped silica and germania glasses free of other dopants: First- principle study, Opt Mater Express, 3(2013)1059-1074.
Asian Journal of Physics |
Vol. 28 Nos 7-9 (2019) 487-498 |
Light propagation through graded-index fibers: Impact of self-imaging on the nonlinear phenomena
Govind P Agrawal
The Institute of Optics,University of Rochester,
Rochester, NY,14627,USA
Invited paper dedicated to Professor Ajoy Ghatak on his 80th Birthday
Periodic self-imaging of optical beams inside graded-Index (GRIN) fibers has been known for more than 45 years. It has been found in recent years that spatial self-imaging also affects the nonlinear temporal evolution of optical pulses inside such fibers. In a 1974 paper that I coauthored with Prof Ghatak [1], it was shown that, in the absence of the nonlinear effects, the optical field at any point inside the GRIN fiber can be written in an analytic form without any explicit reference to its modes. The result was in the form of a propagation kernel that reproduced the input field precisely in a periodic fashion along the length of the GRIN fiber (self-imaging property).We apply this kernel first to a Gaussian beam and discuss how self-imaging affects the nonlinear effects such as modulation instability and supercontinuum generation. We then consider the impact of the spatial shape and position of the input beam (at the input facet of the fiber) on the nonlinear effects by considering off-center launch of a Gaussian beam and a circular beam with uniform intensity. The results show that the results obtained in our 1974 paper are still useful for studying nonlinear optical phenomena in modern GRIN fibers © Anita Publications. All rights reserved.
Keywords: Graded-index fibers, Self-imaging, Nonlinear optics, Modulation instability, Supercontinuum generation
References
1. Agrawal G P, Ghatak A K, Mehta C L, Propagation of a partially coherent beam through Selfoc fibers, Opt Commun, 12(1974)333-337.
2. Jacomme L, Modal dispersion in multimode graded-index fibers, Appl Opt, 14(1975)2578-2584.
3. Agrawal G P, Imaging characteristics of square law media,Nouvelle Revue d’Optique, 7(1976)299-303.
4. Olshansky R, Propagation in glass optical waveguides, Rev Mod Phys, 51(1979)341-367.
5. Iga K, Theory for gradient-index imaging, Appl Opt, 19(1980)1039-1043.
6. Richardson D J, Space-division multiplexing in optical fibres, Nat Photon, 7(2013)354-362.
7. Essiambre R.-J, Ryf R, Fontaine N K, Randel S, Space-division multiplexing in multimode and multicore fibers for high-capacity optical communication, IEEE Photon J, 5(2013)0701307; doi:10.1109/JPHOT.2013.2253091
8. Li G, Bai N, Zhao N, Xia C, Space-division multiplexing: the next frontier in optical communication, Adv Opt Photon, 6(2014)413-487.
9. Mafi A, Pulse propagation in a short nonlinear graded-index multi-mode optical fiber, J Light Wave Technol, 30(2012)2803-2811.
10. Renninger W H, Wise F W, Optical solitons in graded-index multimode fibres, Nat Commun, 4(2013)1719; doi: 10.1038/ncomms2739.
11. Krupa K, Tonello A, Barthélémy A, Couderc V, Shalaby B M, Bendahmane A, Millot G, Wabnitz S, Observation of geometric parametric instability induced by the periodic spatial self-imaging of multimode waves, Phys Rev Lett, 116(2016)183901; doi.org/10.1103/PhysRevLett.116.183901
12. Krupa K, Couderc V, Tonello A, Picozzi A, Barthélémy A, Millot G, Wabnitz S, Spatiotemporal nonlinear dynamics in multimode fibers, in Nonlinear Guided Wave Optics, (Ed ) Wabnitz S, (IOP Science), Chap 14, 2014.
13. Conforti M, Arabi C M, Mussot A, Kudlinski A, Fast and accurate modeling of nonlinear pulse propagation in graded-index multimode fibers, Opt Lett, 42(2017)4004-4007.
14. Wright L G, Ziegler Z M, Lushnikov P M, Zhu Z, Eftekhar M A, Christodoulides D N, Wise F W, Multimode nonlinear fiber optics: massively parallel numerical solver, tutorial, and outlook, IEEE Journal of Selected Topics in Quantum Electronics, 24, 5100516(2018); doi: 10.1109/JSTQE.2017.2779749
15. Ahsan A S, Agrawal G P, Graded-index solitons in multimode fibers, Opt Lett, 43(2018)3345-3348.
16. Agrawal G P, Self-imaging in multimode graded-index fibers and its impact on the nonlinear phenomena, Opt Fiber Technol, 50 (2019)309-316.
17. Ghatak A, Thyagarajan K, An Introduction to Fiber Optics, (Cambridge University Press), 1999.
18. Agrawal G P, Nonlinear Fiber Optics, 6th edn, (Academic Press), 2020.
19. Ponomarenko Sergey A, Self-imaging of partially coherent light in graded-index media, Opt Lett, 40(2015)566-568.
20. Boyd R W, Nonlinear Optics,3 rd edn, (Academic Press), 2008.
21. Karlsson M, Anderson D, Desaix M, Dynamics of self-focusing and self-phase modulation in a parabolic index optical fiber, Opt Lett, 17(1992)22-24.
22. Poletti F, Horak P, Description of ultrashort pulse propagation in multimode optical fibers, J Opt Soc Am B, 25(2008)1645-1654.
23. Longhi S, Modulation in stability and space-time dynamics in nonlinear parabolic-index optical fiber, Opt Lett, 28(2003)2363-2365.
24. Lopez-Aviles H E, Wu F O, Eznaveh Z S, Eftekhar M A, Wise F, Correa R A, Christodoulides D N, Asystematic analysis of parametric instabilities in nonlinear parabolic multimode fibers, APLPhoton, 4, 022803(2019); doi:10.1063/1.5044659
25. Matera F, Mecozzi A, Romagnoli M, Settembre M, Side band instability induced by periodic power variation in long-distance fiber links, Opt Lett, 18(1993)1499-1501.
26. Hasegawa A, Self-confinemen to multimode optical pulse in a glass fiber, Opt Lett, 5(1980)416-417.
27. Crosignani B, Porto P D, Soliton propagation in multimode optical fibers, Opt Lett, 6(1981)329-330.
28. S.-S. Yu, Chien C.-H, Lai Y, Wang J, Spatio-temporal solitary pulses in graded-index materials with Kerr nonlinearity, Opt Commun, 119(1995)167-170.
29. Raghavan S, Agrawal G P, Spatiotemporal solitons in inhomogeneous nonlinear media, Opt Commun, 180(2000)377-382.
30. Kivshar Y S, Agrawal G P, Optical Solitons: From Fibers to Photonic Crystals, (Academic Press), Chap 7, 2003,
31. Lin Q, Agrawal G P, Raman response function for silica fibers, Opt Lett, 31(2006)3086-3088.
32. Wright L G, Wabnitz S, Christodoulides D N, Wise F W, Ultrabroadband dispersive radiation by spatiotemporal oscillation of multimode waves, Phys Rev Lett, 115(2015)223902; doi.org/10.1103/PhysRevLett.115.223902
33. Ahsan A S, Agrawal G P, Spatio-temporal enhancement of Raman-induced frequency shifts in graded-index multimode fibers, Opt Lett, 44(2019)2637-2640.
34. Ahsan A S, Agrawal G P, Effect of beam shape on the nonlinear effects inside a graded-index fiber, J Opt Soc Am B, (submitted).
Asian Journal of Physics |
Vol. 28 Nos 7-9 (2019) 499-508 |
A world without fibre Bragg gratings
Raman Kashyap
Fabulas Laboratory, Department of Physics Engineering,
Department of Electrical Engineering,
Polytechnique Montreal, Montreal, H3T 1J4,Canada
Invited Article dedicated to Prof Ajoy Ghatak on his 80th Birthday
It is hard to imagine what the world of photonics would be today if photosensitivity in optical fibre and fibre Bragg gratings (FBGs) had not been discovered. This article traces the development of FBGs, without which, research and commercialization in many diverse areas, from lasers, sensors to bio-photonics would have been impossible. © Anita Publications. All rights reserved.
Keywords: Fibre Bragg gratings, Fibre lasers, Optical sensors, Bio-photonics, Random lasers, Anderson localization
References
1. Kashyap R, Armitage J R, Wyatt R W, Davey S T & D L Williams, All-fibre narrow-band reflection gratings at 1500nm, Electron Lett, 26(1990)730-731.
2. Hill K O, Fujii Y, Johnson D C, B S Kawasaki B S, Photosensitivity inoptical waveguides: Application to reflection filter fabrication, Appl Phys Lett, 32(1978)647; doi.org/10.1063/1.89881.
3. Yeh P, Yariv A, Marom E, Theory of Bragg fiber, J Opt Soc Am, 68(1978)1196-1201.
4. Martin P J, Oldaker B G, Miklich A H, Pritchard D E, Bragg scattering of atoms from a standing light wave, Phys Rev Lett, 60(1988)515; doi.org/10.1103/PhysRevLett.60.515
5. Ghatak A K, Thyagarajan K, Optical Electronics, (Cambridge University Press), 1989.
6. Kashyap R, Ainslie B J, Maxwell G D, Second-harmonic generation in a GeO2 ridge waveguide, Electron Lett, 25(1989)206-208.
7. Davis K M, Miura K, Sugimoto N, Hirao K, Writing waveguides in glass with a femtosecond laser, Opt Lett, 21(1996)1729-1731.
8. Kashyap Raman, Fiber Bragg Gratings, (Academic Press), 2010.
9. See e.g. https://www.proximion.com.
10. Sugimoto R, Ichii K, Goto R, Kashiwagi M, Kitabayashi T, Shimada N, Masahiro Kashiwagi, 4 kW Continuous Wave Fiber Laser, Fujikura Technical Review No 44, 2015.
11. Hargreaves D, Lick G S, Ventrudo B F, High-power 980-nm pump module operating without a thermoelectric cooler, Optical Fiber Communications Conference, Paper ThG3, 1996.
12. Guan B-O, Tam H-Y, Tao X-M, Dong X-Y, Simultaneous Strain and Temperature Measurement Using a Superstructure Fiber Bragg Grating, IEEE Photon Technol Lett, 12(2000)675-677,
13. Kashyap R, Wyatt R, McKee P F, Wavelength flattened saturated erbium amplifier using multiple side-tap Bragg gratings, Electron Lett, 29(1993)1025-1026.
14. See e.g.www.mpbcommunications.com.
15. See e.g. www.itftechnologies.com
16. Baiad M D, Kashyap R, Concatenation of surface plasmon resonance sensors in a single optical fiber using tilted fiber Bragg gratings, Opt Lett, 40(2015)115-118.
17. Aissa B, Haddad E I, Kruzelecky R V, Jamroz W R, Fibre Bragg Gratings in Harsh and Space Environments: Principles and applications, Materials, Circuits & amp; Devices, (Institution of Engineering and Technology ), 2019, doi: IET Digital Library, https://digital-library.theiet.org/content/books/cs/pbcs069e.
18. Bland-Hawthorn J, Englund M, Edvell G, New approach to atmospheric OH suppression using an aperiodic fibre Bragg grating, Opt Express, 12(2004)5902-5909.
19. Optical Fiber Sensor Technology: Fundamentals, (eds) Grattan K T V, Meggitt B T, (Springer), 2019.
20. Sefati S, Pozin M, Alambeigi F, Iordachita I, Taylor R H, Armand M, A highly sensitive fiber Bragg grating shape sensor for continuum manipulators with large deflections, in 2017 IEEE SENSORS. IEEE, 2017, pp. 1–3.
21. Campbell R J, R Kashyap R, Spectral profile and multiplexing of Bragg gratings in photosensitive fiber, Opt Lett, 16(1991)898-900.
22. Durkin M, Ibsen M, Cole M J, Laming R I, 1m long continuously-written fibre Bragg gratings for combined 2nd and 3rd order dispersion compensation, Electron Lett, 33(1997)1891-1893.
23. See e.g. https://www.nktphotonics.com
24. Perlin V E, Winful H G, Distributed feedback fiber Raman laser, IEEE J Quantum Electron, 37(2001)38-47.
25. Jain R K, Chinlon Lin, Stolen R H, Pleibel W, Kaiser P, A high-efficiency tunable cw Raman oscillator, Appl Phys Lett, 30(1977)162-164.
26. Westbrook P S, Abedin K S, Nicholson J W, Kremp T, Porque J, Raman fiber distributed feedback lasers, Opt Lett, 36(2011)2895-2897.
27. Shi J, Alam S U, Ibsen M, “Highly efficient Raman distributed feedback fibre lasers,” Opt Express, 20(2012) 5082-5091.
28. Shi J, Alam S, Ibsen M, Sub-watt threshold, kilohertz-linewidth Raman distributed-feedback fiber laser, Opt Lett, 37(2012)1544-1546.
29. Gagné M, Loranger S, Lapointe J, Kashyap R, Fabrication of high quality, ultra-long fiber Bragg gratings: up to 2 million periods in phase, Opt Express, 22(2014)387-398.
30. Loranger S, R Kashyap R, Are optical fibers really uniform? Measurement of refractive index on a centimeter scale, Opt Lett, 42(2017)1832-1835, .
31. Gagné M, Kashyap R, Demonstration of a 3 mW threshold Er-doped random fiber laser based on a unique fiber Bragg grating, Opt Express, 17(2009)19067-19074.
32. Anderson P W, Absence of diffusion in certain random lattices, Phys Rev, 109(1958)1492-1505.
33. Gagné M, Kashyap R, Random fiber Bragg grating Raman fiber laser, Opt Lett, 39(2014)2755-2758.
34. Loranger S, Lambin-Iezzi V, Kashyap R, Reproducible ultra-long FBGs in phase corrected non-uniform fibers, Optica, 4(2017)1143-1146.
35. Loranger S, Tehranchi A, Winful H, Kashyap R, Realization and optimization of phase-shifted distributed feedback fiber Bragg grating Raman lasers, Optica, 5(2018)295-302.
36. Lee H, Agrawal G P, Suppression of stimulated Brillouin scattering in optical fibers using fiber Bragg gratings, Opt Express, 11(2003)3467-3472,
37. Abedin K S, Westbrook P S, Nicholson J W, Porque J, Kremp T, Liu X, Single-frequency Brillouin distributed feedback fiber laser, Opt Lett, 37(2012)605-607.
38. Winful H G, Kabakova I V, Eggleton B J, Model for distributed feedback Brillouin lasers, Opt Exp, 21(2013) 16191-16199,
39. Loranger S, Lambin-Iezzi V, Wahbeh M, Kashyap R, Stimulated Brillouin scattering in ultra-long distributed feedback Bragg gratings in standard optical fiber, Opt Lett, 41(2016)1797-1800.
40. Loranger S, Kashyap R, Efficiency increase of distributed feedback Raman fiber lasers by dynamic control of the phase shift, Opt Lett, 43(2018)5705-5708.
41. Karpov V, Loranger S, Kashyap R, A Tunable, Single Frequency, Linearly Polarized DFB Raman Fiber Laser Operating at 1178-nm, Bragg Gratings, Photosensitivity and Poling in Glass Waveguides and Materials, paper BW4A 2, Optical Society of America, 2018.
42. Hu Y, Broderick N G R, Improved design of a DFB Raman fibre laser, Opt Commun, 282(2009)3356-3359.
43. Kremp T, Abedin K S, Westbrook P S, Closed-form approximations to the threshold quantities of distributed-feedback lasers with varying phase shifts and positions, IEEE J Quantum Electron, 49(2013)281-292.
44. Tehranchi A, S. Loranger, and R. Kashyap, Engineered p-Phase-Shifted Fiber Bragg Gratings for Efficient Distributed Feedback Raman Fiber Lasers, IEEE Journal of Quantum Electronics, 54(2018)1-7.
45. Shapira O, Fischer B, Localization of light in a random-grating array in a single-mode fiber, J Opt Soc Am B, 22(2005)2542-2552.
46. Hlil A R, Lima B C, Thomas J, Boisvert J S, Iden H, Garcia-Puente Y, Maia L J Q, Ledemi Y, Messaddeq Y, Gomes A S L, Kashyap R, Rare earth doped PDMS elastomeric random lasers, Opt Mater, 97(2019)109387; doi.org/10.1016/j.optmat.2019.109387
47. Tehranchi A, Iezzi V L, Kashyap R, Power Fluctuations and Random Lasing in Multiwavelength Brillouin Erbium-Doped Fiber Lasers, J Lightwave Technol, 37(2019)4439-4444.
48. Lima B C, Pincheira P I R, Moura A L, Gomes A S L, Menezes L de S, Raposo E P, Araújo C B de, Kashyap R, Fluctuations Statistics in an Erbium Random Fiber Laser, OSA Frontiers in Optics JW3A. 73, 2018; doi.org/10.1364/FIO.2018.JW3A.73.
49. Lima B C, Pincheira P I R, Raposo E P, Menezes L de S, Araújo C B de, Gomes A S L, Kashyap R, Extreme-value statistics of intensities in a cw-pumped random fiber laser, Phys Rev A, 96 (2017)013834; doi.org/10.1103/PhysRevA.96.013834
50. Lima B C, Gomes A S L, Pincheira P I R, Moura A L, Gagné M, Raposo E P, Araújo C B de, Kashyap R, Observation of Lévy statistics in one-dimensional erbium-based random fiber laser, J Opt Soc Am B, 34(2017)293-299.
51. Gonzalez I R R, Lima B C, Pincheira P I R, Brum A A, Macedo A M S, Vasconcelos G L, Menezes L de S, Raposo E P, Gomes A S L, Kashyap R, Turbulence hierarchy in a random fibre laser, Nat Commun, 8(2017)15731; doi.org/10.1038/ncomms15731
52. Gomes A S L, Lima B C, Pincheira P I R, Moura A L, Gagné M, Raposo E P, Araújo C B de, Kashyap R, Glassy behavior in a one-dimensional continuous- wave erbium-doped random fiber laser, Phys Rev A, 94(2016) 011801(R); doi.org/10.1103/PhysRevA.94.011801
53. Lizárraga N, Puente N P, Chaikina E I, Leskova T A, Méndes E R, Single-mode Er-doped fiber random laser with distributed Bragg grating feedback, Opt Express, 17(2009)395-404.
54. Clivaz X, Marquis-Weible F, Salathe R P, Novak R P, Gilgen H H, High-resolution reflectometry in biological tissues, Opt Lett, 17(1992)4-6.
55. Froggatt M, Moore J, High-spatial-resolution distributed strain measurement in optical fiber with Rayleigh scatter, Appl Opt, 37(1998)1735-1740.
56. Loranger S, Mathieu Gagné, Victor Lambin-Iezzi, Raman Kashyap, Rayleigh scatter-based order of magnitude increase in distributed temperature and strain sensing by simple UV exposure of optical fibre, Nature Sci Rep, 5(2015)11177; doi: 10.1038/srep11177
57. Parent F, Loranger S, Mandal K K, LambinIezzi V, Lapointe J, Boisvert J-S, Baiad M D, Kadoury S, Kashyap R, Enhancement of accuracy in shape sensing of surgical needles using optical frequency domain reflectometry in optical fibers, Biomed Opt Express, 8(2017)2210-2221.
58. Mandal K, Parent F, Martel S, Kashyap R, Kadoury S, Vessel-based registration of an optical shape sensing catheter for M R navigation, Int J CARS, 11(2016)1025-1034.
59. Monet F, Loranger S, Lambin-Iezzi V, Drouin A, Kadoury S, Kashyap R, The ROGUE: a novel, noise-generated random grating, Opt Express, 27(2019)13895-13909.
60. Lorre P, Monet F, Gauthier M, Poiffaut A, Roberge A, Kadoury S, R Kashyap R, Extruded optical fiber triplets for 3D shape sensing for minimally invasive surgery, Seventh European Workshop on Optical Fibre Sensors, Vol 11199, 111991L, International Society for Optics and Photonics, 2019.
Asian Journal of Physics |
Vol. 28 Nos 7-9 (2019) 509-516 |
Twisted light: beams carrying orbital angular momentum
Siddharth Ramachandran
Boston University,
8 Saint Mary’s Street, Boston, MA 02215, USA
Invited article dedicated to Prof Ajoy Ghatak on his 80th Birthday
Light can carry both orbital angular momentum (OAM) and spin angular momentum (SAM), related to wavefront rotation and polarization, respectively. The ability to exploit these degrees of freedom in so-called optical vortices has spawned applications across the fields of opto-mechanics, sensing, telecommunications and manufacturing . In free space or bulk-optic media, SAM and OAM represent independent quantum numbers. However, from atomic and molecular systems, we also know that the presence of a confinement potential yields a spin-orbit interaction Hamiltonian that hybridises these states. Photonic analogues of such spin-orbit interactions are now accessible in optical fibers, since an optical waveguide is essentially a confining “potential” for light. Here, we will show how generation, stable propagation and nonlinear interactions of light beams containing phase or polarization singularities are both altered by, and, from a device perspective, benefit from engineering spin-orbit interactions of light via optical waveguide design. After elucidating the fundamental properties of these exotic modes, we will review recent applications that have exploited fiber OAM modes. © Anita Publications. All rights reserved.
Keywords: Orbital angular momentum; Spin angular momentum; Optical vortices; Lasers; Nonlinear optics; Imaging; Sensing
References
1. Mandel L, Wolf E, Optical Coherence and Quantum Optics, (Cambridge University Press), 1955.
2. Beth R A, Phys Rev, 50(1936)115-125.
3. Allen L, Beijersbergen M, Spreeuw R, Woerdman J, Orbital angular momentum of light and the transformation of Laguerre-Gaussian laser modes, Phys Rev A, 45(1992)8185-8188
4. Gibson G, Courtial J, Padgett M, Vasnetsov M, Pas’ko V, Barnett S, Franke-Arnold S, Free-space information transfer using light beams carrying orbital angular momentum, Opt Express, 12(2004)5448-5456.
5. Wang J, Yang J.-Y, Fazal I M, Ahmed N, Yan Y, Huang H, Ren Y, Yue Y, Dolinar S, Tur M, Willner A E, Terabit free-space data transmission employing orbital angular momentum multiplexing, Nature Photonics, 6(2012)488-496.
6. Huang H, Xie G, Yan Y, Ahmed N, Ren Y, Yue Y, Rogawski D, Willner M J, Erkmen B I, Birnbaum K M, Dolinar S J, Lavery M P J, Padgett M J, Tur M, Willner A E, 100 Tbit/s free-space data link enabled by three-dimensional multiplexing of orbital angular momentum, polarization, and wavelength, Opt Lett, 39(2014)197-200.
7. Mair A, Vaziri A, Weihs G, Zeilinger A, Entanglement of the orbital angular momentum states of photons, Nature, 412(2001)313-316.
8. Vaziri A, Weihs G, Zeilinger A, Experimental two-photon, three dimensional entanglement for quantum communication, Phys Rev Lett, 89(2002)240401; doi.org/10.1103/PhysRevLett.89.240401
9. He H, Friese MEJ, Heckenberg N R, Rubinsztein-Dunlop H, Direct observation of transfer of angular momentum to absorptive particles from a laser beam with a phase singularity, Phys Rev Lett, 75(1995)826-829.
10. Simpson N B, Dholakia K, Allen L, Padgett M J, Mechanical equivalence of spin and orbital angular momentum of light: an optical spanner, Opt Lett, 22(1997)52-54.
11. Curtis J E, Grier D G, Modulated optical vortices. Opt Lett, 28(2003)872-874.
12. Kreysing M K, Kießling T, Fritsch A, Guck J R, Josef A K, The optical cell rotator. Opt Express, 16(2008)912-914.
13. Ladavac K, Grier D, Microoptomechanical pumps assembled and driven by holographic optical vortex arrays. Opt Express, 12(2004)1144-1149.
14. Babiker M, Power W L, Allen L, Light-induced torque on moving atoms, Phys Rev Lett, 73(1994)1239-1242.
15. Madison K W, Chevy F, Wohlleben W, Dalibard J, Vortex formation in a stirred Bose–Einstein condensate, Phys Rev Lett, 84(2000)806-809.
16. Zhan Q, Cylindrical vector beams: from mathematical concepts to applications, Adv Opt Photon, 1(2009)1-57.
17. Dorn R, Quabis S, Leuchs G, Shaper focus for a radially polarized light beam, Phys Rev Let, 91(2003)233901; doi.org/10.1103/PhysRevLett.91.233901
18. Salamin Y I, Electron acceleration from rest in vacuum by an axicon Gaussian laser beam, Phys Rev A, 73(2006) 43402; doi.org/10.1103/PhysRevA.73.043402
19. Zhan Q, Trapping metallic Rayleigh particles with radial polarization, Opt Express, 12(2004)3377-3382.
20. Novotny L, Beversluis M R, Youngworth K S, Brown T G, Longitudinal Field Modes Probed by Single Molecules, Phys Rev Lett, 86(2001)5251; doi.org/10.1103/PhysRevLett.86.5251
21. Nesterov A V, Niziev V G, Laser beams with axially symmetric polarization, J Phys D, 33(2000)1817; doi.org/10.1088/0022-3727/33/15/310
22. Hell S W, Wichmann J, Opt Lett,19(194)780-782.
23. Vitullo D L, Leary C C, Gregg P, Smith R A, Reddy D V, Ramachandran S, Raymer M G, Observation of interaction of spin and intrinsic orbital angular momentum of light, Phys Rev Lett, 118(2017)083601; doi.org/10.1103/PhysRevLett.118.083601.
24. Golowich S, Ramachandran S, Impact of fiber design on polarization dependence in microbend gratings, Opt Express, 13(2005)6870-6877.
25. Ramachandran S, Golowich S, Yan M F, Monberg E, Dimarcello F V, Fleming J, Ghalmi S, Wisk P, Lifting polarisation degeneracy of modes by fiber design: a platform for polarisation insensitive microbend fiber gratings, Opt Lett, 30(2005)2864-2866.
26. Noda J, Okamoto K, Sasaki Y, Polarization-maintaining fibers and their applications, J Lightwave Technol, 4(1986)1071-1089.
27. Ramachandran S, Kristensen P, Optical vortices in fiber, Invited Paper, J Nanophotonics, 2(2013)455; doi.org/10.1515/nanoph-2013-0047.
28. Bozinovic N, Yue Y, Ren Y, Tur M, Kristensen P, Huang H, Willner A E, Ramachandran S, Terabit-scale orbital angular momentum mode division multiplexing in fibers, Science, 340(2013)1545-1548.
29. Nejad R M, Allahverdyan K, Vaity P, Amiralizadeh S, Brunet C Messaddeq Y, LaRochelle S, Rusch L A, Mode Division Multiplexing Using Orbital Angular Momentum Modes Over 1.4-km Ring Core Fiber, J Lightwave Technol, 34(2016)4252-4258.
30. Wang L, Nejad R M, Corsi A, Lin J, Messaddeq Y, Rusch L, LaRochelle S, Linearly polarized vector modes: enabling MIMO-free mode-division multiplexing. Opt Express, 25(2017)11736-11749.
31. Ingerslev K,Gregg P, Galili M, Ros F D, Hu H, Bao F, Castaneda M A U, Kristensen P, Rubano A, Marrucci L, Rottwitt K, Morioka T, Ramachandran S, Oxen L K, 12 mode, WDM, MIMO-free orbital angular momentum transmission, Opt Express, 26(2018) 20225-20232.
32. Sit A, Fickler R, Alsaiari F, Bouchard F, Larocque H, Gregg P, Yan L, Boyd R W, Ramachandran S, Karimi E, Quantum cryptography with structured photons through a vortex fiber, Opt Lett, 43(2018)4108-4111.
33. Cozzolino D, Bacco D, Lio B D, Ingerslev K, Ding Y, Dalgaard K, Kristensen P, Galili M, Rottwitt K, . Ramachandran S, Oxenløwe L K, Orbital Angular Momentum States Enabling Fiber-based High-dimensional Quantum Communication, Phys Rev Appl, 11(2019) 064058 ; doi.org/10.1103 /PhysRevApplied.11.064058
34. Yan L, Kristensen P, Ramachandran S, Vortex fibers for stimulated emission depletion (STED) microscopy, APL Photonics, 4(2019)022903; doi.org/10.1063 /1.5045233
35. Heffernan B M, Meyer S A, Restrepo D, Siemens M E, Gibson E A, Gopinath J T, A Fiber-Coupled Stimulated Emission Depletion Microscope for Bend- Insensitive Through-Fiber Imaging, Sci Rep, 9(2019)11137; doi: 10.1038/s41598-019-47319-w
36. Prabhakar G, Gregg P, Rishoj L, Kristensen P, Ramachandran S, Octave-wide supercontinuum generation of light-carrying orbital angular momentum, Opt Express, 27(2019)11547-11556.
37. Steven Golowich, Asymptotic theory of strong spin–orbit coupling in optical fiber, Opt Lett, 39(2014) 92-95.
38. Götte J B, O’Holleran K, Preece D, Flossmann F, Franke-Arnold S, Barnett Stephen M, Padgett M J, Light beams with fractional orbital angular momentum and their vortex structure, Opt Express, 16(2008)993-1006.
39. Gregg P, Kristensen P, Rubano A, Golowich S, Marrucci L, Ramachandran S, Enhanced Spin Orbit Interaction of Light in Highly Confining Optical Fibers for Mode Division Multiplexing, Nat Commun, to appear. [In Press]
Asian Journal of Physics |
Vol. 28 Nos 7-9 (2019) 517-526 |
High-efficiency ring similariton fiber laser with nonlinear spectral compression
A S Abramov1, D A Korobko1, I O Zolotovskii1 and A A Sysoliatin1,2
1Ulyanovsk State University, 42 Leo Tolstoy Street, Ulyanovsk, 432970, Russian Federation
2General Physics institute, 38, Vavilov Street, Russian Federation
Invited Article dedicated to Prof Ajoy Ghatak on his 80th Birthday
We present a theoretical model of a ring similariton fiber laser employing nonlinear spectrum compression. A pair of tunnel-coupled optical fibers is used for passive mode-locking. The laser characteristics are simulated for a wide range of laser operation regimes and configuration parameters. The optimized laser system design enables generation of pulses with the maximal efficiency and spectral density providing the lowest losses of energy inside the cavity. © Anita Publications. All rights reserved.
Keywords: Ring fiber laser, Normal dispersion, Similariton, Spectral compression
References
1. Fu W, Wright L G, Sidorenko P, Backus S, Wise F W, Several new directions for ultrafast fiber lasers, Opt Express, 26(2018)9432-9463.
2. Renninger W H, Chong A, Wise F W, Giant-chirp oscillators for short-pulse fiber amplifiers, Opt Lett, 33(2008)3025-3027.
3. Chong A, Renninger W H, Wise F W, All-normal-dispersion femtosecond fiber laser with pulse energy above 20 nJ, Opt Lett, 32(2007)2408-2410.
4. Ruehl A, Kuhn V, Wandt D, Kracht D, Normal dispersion erbium-doped fiber laser with pulse energies above 10 nJ, Opt Express, 16(2008)3130-3135.
5. Wise F W, Chong A, Renninger W H, High-energy femtosecond fiber lasers based on pulse propagation at normal dispersion, Laser Photon Rev, 2(2008)58-73.
6. Lecaplain C, Chédot C, Hideur A, Ortaç B, Limpert J, High-power all-normal-dispersion femtosecond pulse generation from a Yb-doped large-mode-area microstructure fiber laser, Opt Lett, 32(2007)2738-2740.
7. Chong A, Wright L G, Wise F W, Ultrafast fiber lasers based on self-similar pulse evolution: a review of current progress, Rep Prog Phys, 78(2015)113901; doi.org/10.1088/0034-4885/78/11/113901
8. Zolotovskii I O, Korobko D A, Stoliarov D A, Fibre laser system providing generation of frequency-modulated pulses with a spectral width exceeding the gain linewidth, Quantum Electron, 46(2016)1092; doi.org/10.1070/QEL16218
9. Renninger W H, Chong A, Wise F W, Self-similar pulse evolution in an all-normal-dispersion laser, Phys Rev A, 82(2010) 021805(R); /doi.org/10.1103
/PhysRevA.82.021805
10. Nie B, Pestov D, Wise F W, Dantus M, Generation of 42-fs and 10-nJ pulses from a fiber laser with self-similar evolution in the gain segment, Opt
Express, 19(2011)12074-12080.
11. Krylov A A, Sazonkin S G, Lazarev V A, Dvoretskiy D A, Leonov S O, Pnev A B, Dianov E M, Ultra-short pulse generation in the hybridly mode-locked erbium-doped all-fiber ring laser with a distributed polarizer, Laser Phys Lett, 12(2015)065001; doi.org/10.1088/1612-2011/12/6/065001
12. Tang Y, Liu Z, Fu W, Wise F W, Self-similar pulse evolution in a fiber laser with a comb-like dispersion-decreasing fiber, Opt Lett, 41(2016)2290-2293.
13. Bale B G, Wabnitz S, Strong spectral filtering for a mode-locked similariton fiber laser, Opt Lett, 35(2010)2466-2468.
14. Stolen R H, Lin Q, Self-phase modulation in silica optical fibers, Phys Rev A, 17(1978)1448-1453.
1 5. Limpert J P, Gabler T, Liem A, Zellmer H, Tünnermann A, SPM-induced spectral compression of picosecond pulses in a single-mode Yb-doped fiber amplifier, Appl Phys B, 74(2002)191-195.
16. Andresen E R, Dudley J M, Oron D, Finot C, Rigneault H, Transform-limited spectral compression by self-phase modulation of amplitude-shaped pulses with negative chirp, Opt Lett, 36(2011)707-709.
17. Fatome J, Kibler B, Andresen E R, Rigneault H, Finot C, All-fiber spectral compression of picosecond pulses at telecommunication wavelength enhanced by amplitude shaping, Appl Opt, 51(2012)4547-4553.
18. Korobko D A, Okhotnikov O G, Zolotovskii I O, Multistage fiber preamplifier employing spectral compression for generation of high-energy laser pulses, J Opt Soc Am B, 33(2016)239-245.
19. Wynands R, Coste O, Rembe C, Meschede D, How accurate is optical second-harmonic generation ?, Opt Lett, 20(1995)1095-1097.
20. Makarov G N, Low energy methods of molecular laser isotope separation, Physics-Uspekhi, 58(2015)670-700.
21. Boscolo S, Turitsyn S K, Finot C, Amplifier similariton fiber laser with nonlinear spectral compression, Opt Lett, 37(2012)4531-4533.
22. Walton D T, Winful H G, Passive mode locking with an active nonlinear directional coupler: positive group-velocity dispersion, Opt Lett, 18(1993)720-722.
23. Agrawal G P, Nonlinear fiber optics,4th edn, (Springer), 2007.
24. Chen C J, Wai P K A, Menyuk C R, Soliton fiber ring laser, Opt Lett, 17(1992)417-419.
25. Finot C, Boscolo S, Design rules for nonlinear spectral compression in optical fibers, J Opt Soc Am B , 33(2016)760-767.
26. Abramov A S, Zolotovskii I O, Korobko D A, Fotiadi A A, A fiber-optic system generating pulses of high spectral density, Opt and Spectrosc, 124(2018)343-348.
27. Dudley J M, Finot C, Richardson D J, Millot G, Self-similarity in ultrafast nonlinear optics, Nature Phys, 3(2007)597-603.
28. Boscolo S, Finot C, Karakuzu H, Petropoulos P, Pulse shaping in mode-locked fiber lasers by in-cavity spectral filter, Opt Lett, 39(2014)438-441.
29. Zolotovskii I O, Korobko D A, Sysoliatin A A, Fotiadi A A, Multistage fiber amplifier with spectral compression elements for high-energy laser pulse generation, J Rus Laser Res, 37(2016)448-458.
30. Monmayrant A, Weber S, Chatel B, A newcomer’s guide to ultrashort pulse shaping and characterization, J Phys B: At Mol Opt Phys, 43(2010) 103001; doi.org/10.1088/0953-4075/43/10/103001
Asian Journal of Physics |
Vol. 28 Nos 7-9 (2019) 529-537 |
Blending random lasers and second order nonlinearities in
neodymium-doped crystalline powders
Anderson S L Gomes1, Lauro J Q Maia2, André L Moura3 and Cid B de Araújo1
1Departamento de Física, Universidade Federal de Pernambuco, 50670-901, Recife, PE, Brazil
2Grupo Física de Materiais, Instituto de Física, Universidade Federal de Goiás, 74001-970, Goiânia-GO, Brazil
3Grupo de Física da Matéria Condensada, Núcleo de Ciências Exatas – NCEx, Campus Arapiraca,
Universidade Federal de Alagoas, 57309-005, Arapiraca, AL, Brazil
Invited Article dedicated to Prof Ajoy Ghatak on his 80th Birthday
The advent of the LASER in 1960, and its further development in the subsequent decades, radically changed the way light-matter interaction was investigated, opening new field of studies and applications based on optics and photonics. To achieve the LASER unique temporal, spectral and spatial coherence, a gain medium is placed between two fixed mirrors and appropriately excited. The so-called laser cavity provides the required feedback to achieve coherent laser oscillation. Due to its high intensity, when the laser light is propagated through a non-centrosymmetric crystal, its fundamental frequency can be doubled, leading to the so-called second harmonic generation. The observation of such phenomena in 1961 paved the way to the field of nonlinear optics. However, in 1967 a new idea was proposed that coherent radiation could also be obtained in a gain medium without the need for a fixed optical cavity. The required feedback to sustain LASER oscillation would arise from a scattering medium. In this review article, we will briefly describe the basis of random lasers and random fiber lasers, and how they can benefit from second order nonlinear optical processes, namely self-second harmonic generation and sum-frequency generation in the same laser medium. Therefore, tunable multi-wavelength emission not easily achievable in random lasers was demonstrated. © Anita Publications. All rights reserved.
Keywords: Lasers, Random lasers, Random fiber laser, Nonlinear optics
References
1. Letokhov V S, Stimulated emission of an ensemble of scattering particles with negative absorption, ZhETF Pisma Redaktsiiu, 5(1967)262-265 Russian. JETP Lett, 5(1967)212-215.
2. Letokhov V S, Generation of light by a scattering medium with negative resonance absorption, Zh Exp and Teor Fiz, 53(1968)442-452; Russian. Sov Phys JETP, 26(1968)835-840.
3. Markushev V M, Zolin V F, Briskina Ch M, Luminescence and stimulatedemission of neodymium in sodium lanthanum molybdate powders, Sov J Quantum Electron, 16(1986)28-283.
4. Markushev V M, Ter-Gabrélyan N É, Briskina Ch M, Velan, V R, Zolin V F, Stimulated emission kinetics of neodymium powder lasers, Sov J Quantum Electron, 20(1990)773-777.
5. Gouedard C, Husson D, Sauteret C, Auzel F, Migus A, Generation of spatially incoherent short pulsesin laser pumped neodymium stoichiometric crystals and powders, J Opt Soc Am B, 10(1993)2358-2363.
6. Lawandy N M, Balachandran R M, Gomes A S L, Sauvain E, Laser action in strongly scattering media, Nature, 368(1994)436-438.
7. Luan F, Gu B, Gomes A S L, Yong K-T, Wen S, Prasad P N, Lasing in Nanocomposite Random Media, Nano Today, 10(2015)168-192.
8. Cao H, Zhao Y G, Ho S T, Seelig, Wang Q W, Chang R P H, Random laser action in semiconductor powder, Phys Rev Lett, 82(1999)2278-2281.
9. Noginov M A, Solid-State Random Lasers, (Springer, Berlin), 2005.
10. Matos C J S de, Menezes L de S, Brito-Silva A M, Martinez Gámez M A, Gomes A S L, Araújo C B de, Random Fiber Laser, Phys Rev Lett, 99(2007)1539034; doi.org/10.1103/PhysRevLett.99.153903
11. Gagné M, Kashyap R, Demonstration of a 3mW threshold Er-doped random fiber laser based on a unique fiber Bragg grating, Opt Express, 17(2009)19067-19074.
12. Turitsyn S K, Babin S A, El-Taher A E, Harper P, Churkin D V, Kablukov S I, Ania-Castanon J D, Karalekas V, Podivilov E V, Random distributed feedback fibre laser, Nat Photonics, 4(2010)231-235.
13. Wang Z, Wu H, Fan M, Zhang L, Rao Y, Zhang W, Jia Xinhong, High Power Random Fiber Laser with Short Cavity Length: Theoretical and Experimental Investigations, IEEE J Sel Top in Quant Electron, 21(2015)0900506; doi. 10.1109/JSTQE.2014.2344293
14. Zhang H, Huang L, Song J, Wu H, Zhou P, Wang X, Wu J, Xu J, Wang Z, Xu X, Rao Y, Quasi-kilowatt random fiber laser, Opt Lett, 44(2019)2613-2616.
15. Pang M, Bao X, Chen L, Observation of narrow linewidth spikes in the coherent Brillouin random fiber laser, Opt Lett, 38(2013)1866-1868.
16. Churkin D V, Sugavanam S, Vatnik I D, Wang Z, Podivilov E V, Babin S A, Rao Y, Turitsyn S K, Recent advances in fundamentals and applications of random fiber lasers, Adv Opt Photonics, 7(2015)516-569 .
17. Boyd R W, Nonlinear Optics, third Edn, (Academic Press), 2008.,
18. Moura A L, Maia L J Q, Gomes A S L, de Araújo C B, Optimal performance of NdAl3(BO3)4 nanocrystals random lasers, Opt Mater, 62(2016)593-596.
19. de Oliveira M A S, de Araújo C B, Messaddeq Younes, Upconversion ultraviolet random lasing in Nd3+ doped fluoroindate glass powder, Opt Express, 19(2011)5620-5626.
20. Moura A L, Fewo S I, Carvalho M T, Kuzmin A N, Prasad P N, Gomes A S L, de Araújo C B, Random lasing in Nd3+ doped potassium gadolinium tungstate crystal powder, J Appl Phys, 117(2015)083102; doi.org/10.1063/1.4913390
21. Moura A L, Jerez V, Maia L J Q, Gomes A S L, de Araújo C B, Multi-wavelength emission through self-induced second-order wave-mixing processes from a Nd3+ doped crystalline powder random laser, Sci Rep, 5(2015)13816; doi.org/10.1038/srep13816
22. Cao H, Ling Y, Cao C Q, Photon statistics of random lasers with resonant feedback, Phys Rev Lett, 86(2001) 4524-4527.
23. Redding B, Choma M A, Cao H, Speckle-free laser imaging using random laser illumination, Nature Photon, 6(2012)355-359.
24. Barredo-Zuriarrain M, Iparraguirre I, Fernández J, Azkargorta J, Balda R, Speckle-free near-infrared imaging using a Nd3+ random laser, Laser Phys Lett, 14(2017)106201; doi.org/10.1088/1612-202X/aa7874
25. Azkargorta J, Iparraguirre I, Fernández J, Balda R, García-Revilla S, Barredo-Zurriarrain M, Random laser properties of Nd3+ crystal powders, Opt Express, 26(2018)11787-11803.
26. Carreño S J M, Moura A L, Fabris Z V, Maia L J Q, Gomes A S L, de Araújo C B, Interplay between random laser performance and self-frequency conversions in NdxY1.00–xAl3(BO3)4 nanocrystals powders, Opt Mater, 54 (2016)262-268.
27. Moura A L, Carreño S J M, Pincheira P I R, Fabris Z V, Maia L J Q, Gomes A S L, de Araújo C B, Tunable ultraviolet and blue light generation from Nd:YAB random laser bolstered by second-order nonlinear processes, Sci Rep, 6(2016)27107; doi.org/10.1038/srep27107
28. Moura A L, Maia L J Q, Jerez V, Gomes A S L, de Araújo C B, Random laser in Nd:YBO3 nanocrystalline powders presenting luminescence concentration quenching, J Lumin, 214(2014)116543; doi.org/10.1016/j.jlumin.2019.116543
29. Rocha E G, Paz I R S, Santos B J, Soares W C, Lima W de, Leão L M O S, Maia L J Q, Moura A L, Self-induced optical parametric amplification of random laser emission, Laser Phys, 29(2019) 045402; doi.org/10.1088/1555-6611/ab0175
30. de Araújo C B, Gomes A S L, Raposo E P, (Review) Lévy Statistics and Glassy Behavior of Light in Random Fiber Lasers, Appl Sci, 7(2017)644; doi:10.3390/app7070644
Asian Journal of Physics |
Vol. 28 Nos 7-9 (2019) 541-552 |
Towards stable delivery of high energy through background-guided similariton pulses
Piyali Biswas and Somnath Ghosh
Department of Physics
Indian Institute of Technology Jodhpur-342 037, Rajasthan, India
Invited Paper dedicated to Prof A Ghatak on his 80th birthday
Stable delivery of high energy optical pulses through fibers is an attractive field of research owing to its wide applications in defense, bio-medical industry, chemical industry and so on. Delivery of such high energy can be possible by exploring the inherent potential of background-guided parabolic optical pulses. Asymptotic evolution of parabolic pulses largely depends on the initial pulse energy fed at the input of the fiber. Here, we have investigated the effect of presence of a hyperbolic tangent pulse as the background of an input Gaussian pulse. Further, we have numerically demonstrated the stable propagation of the evolved parabolic pulse with its characteristic linear chirp. A background-guided Gaussian pulse and a background-guided hyperbolic secant pulse, centered at 980 nm wavelength, have been fed at the input end of a dispersion decreasing Bragg fiber and allowed to propagate over several kilometers of the fiber length. The entire propagation has come out to be more stable, and shows closer asymptotic behavior in comparison with the conventional propagation of a single Gaussian or a single hyperbolic secant pulse of same energy. Such a scheme should be attractive in stable delivery of high energy pulses including applications in mid-IR spectroscopy, defense and medical surgery. © Anita Publications. All rights reserved.
Keywords: Parabolic pulse, Nonlinear fiber optics, High energy pulse, Similaritons.
References
1. Tomlinson W J, Stolen R H, Johnson A M, Optical wavebreaking of pulses in nonlinear optical fibers, Opt Lett, 10(1985)457-459.
2. Anderson D, Desaix M, Karlsson M, Lisak M, Quiroga-Teixeiro M L, Wave-breaking-free pulses in nonlinear optical fibers, J Opt Soc Am B, 10(1993)118 -1190.
3. Fermann M E, Kruglov V I, Thomsen B C, Dudley J M, Harvey J D, Self-similar propagation and amplification of parabolic pulses in optical fibers, Phys Rev Lett, 84(2000)6010-6013.
4. Kruglov V I, Peacock A C, Harvey J D, Exact self-similar solutions of the generalized nonlinear Schrödinger equation with distributed coefficients, Phys Rev Lett, 90(2003)113902; doi.org/10.1103/PhysRevLett.90.113902
5. Serkin V N, Hasegawa A, Belyaeva T L, Nonautonomous solitons in external potentials, Phys Rev Lett, 98(2007)074102; doi.org/10.1103 /PhysRevLett.98.074102
6. Ponomarenko S A, Agrawal G P, Interactions of chirped and chirp-free similaritons in optical fiber amplifiers, Opt Express, 15(2007)2963-2973.
7. Ponomarenko S A, Agrawal G P, Do soliton like self-similar waves exist in nonlinear optical media?, Phys Rev Lett, 97(2006)013901; doi.org/10.1103 /PhysRevLett.97.013901
8. Kruglov V I, Peacock A C, Harvey J D, Dudley J M, Self-similar propagation of parabolic pulses in normal-dispersion fiber amplifiers, J Opt Soc Am B, 19(2002)461-469.
9. Finot C, Millot G, Billet C, Dudley J M, Experimental generation of parabolic pulses via Raman amplification in optical fiber, Opt Express, 11(2003)1547-1552.
10. Ilday F Ö, Buckley J R, Clark W G, Wise F W, Self-similar evolution of parabolic pulses in a laser, Phys Rev Lett, 92(2004)213902; doi.org/10.1103 /PhysRevLett.92.213902 .
11. Finot C, Millot G, Dudley J M, Asymptotic characteristics of parabolic similariton pulses in optical fiber amplifiers, Opt Lett, 29(2004)2533-2535.
12. Hirooka T, Nakazawa M, Parabolic pulse generation by use of a dispersion-decreasing fiber with normal group velocity dispersion, Opt Lett, 29(2004)498-500.
13. Biswas P, Adhikary P, Biswas A, Ghosh S, Formation and stability analysis of parabolic pulses through specialty microstructured optical fibers at 2.1 mm, Opt Commun, 377(2016)120-127.
14. Finot C, Barviau B, Millot G, Guryanov A, Sysoliatin A, Wabnitz S, Parabolic pulse generation with active or passive dispersion decreasing optical fibers, Opt Exp, 15(2007)15824-15835.
15. Lavdas S, Driscoll J B, Jiang H, Grote R R, Osgood R M, Panoiu N C, Generation of parabolic Similaritons in tapered silicon photonic wires: Comparison of pulse dynamics at telecom and mid-infrared wavelengths, Opt Lett, 38(2013)3953-3956.
16. Finot C, Provost L, Petropoulos P, Richardson D J, Parabolic pulse generation through passive nonlinear pulse reshaping in a normally dispersive two segment fiber device, Opt Express, 15(2007)852-864.
17. Iakushev S O, Shulika O V, Sukhoivanov I A, Passive nonlinear reshaping towards parabolic pulses in the steady-state regime in optical fibers, Opt Commun, 285(2012)4493-4499.
18. Finot C, Dudley J M, Kibler B, Richardson D J, Millot G, Optical parabolic pulse generation and applications, IEEE J Quantum Electron, 45(2009)1482-1489.
19. A C, Wright L, Wise F, Ultrafast fiber lasers based on self-similar pulse evolution: a review of current progress, Rep Prog Phys, 78(2015)113901; doi.org/10.1088/0034-4885/78/11/113901
20. Biswas P, Pal B P, Biswas A, Ghosh S, Toward self-similar propagation of optical pulses in a dispersion tailored, nonlinear, and segmented Bragg-fiber at 2.8 μm, IEEE Photonics J, 9(2017)1-12.
21. Joannopoulos J D, Johnson S G, Winn J N, Meade R D, Photonic Crystals Molding the Flow of Light, (Princeton University Press), 2008.
22. Dasgupta S, Pal B P, Shenoy M R, Nonlinear spectral broadening in solid-core Bragg fibers, J Lightwave Technol, 25(2007)2475-2481.
23. Agrawal S, Nonlinear Fiber Optics, (Academic Press), 2007.
24. Bracun D, Selak L, Optical probing for cnc machining of large parts made from fiber-reinforced polymer composite materials, The Int J Adv Manuf Technol, 100(2019)1855-1865.
25. Ilev I K, Waynant R W, Mid-infrared Semiconductor Optoelectronics, (Springer London, London), chap. Mid-infrared Biomedical Applications, 2006, pp 615-634.
26. Curtis A, Banishev A, Shaw W, Dlott D, Laser-driven flyer platesfor shock compression science: Launch and target impact probed by photon doppler velocimetry, Rev Sci Instruments, 85, 043908(2014); doi.org/10.1063/1.4871361.
27. Nakajima Y, Nishiyama A, Minoshima K, Mode-filtering technique based on all-fiber-based external cavity for fiber-based optical frequency comb, Opt Express, 26(2018)4656-4664.
Towards stable delivery of high energy through background-guided similariton pulses.pdf
Piyali Biswas and Somnath Ghosh
Asian Journal of Physics |
Vol. 28 Nos 7-9 (2019) 555-566 |
Fibre optic sensors – an exemplar on the routes to applications
Brian Culshaw
University of Strathclyde, Glasgow, Scotland UK
Invited Article dedicated to Prof Ajoy Ghatak on his 80th Birthday
Photonics excites as a science and also has very wide-ranging applications potential. The fascination has stimulated extensive academic research, much with intriguing results and often these results offer the hint of potential application. Indeed, many authors emphasise this in their research publications. This application potential, whilst acknowledged through many international organisations and funding bodies, requires substantial commitment to ensure that this potential is realised. The steps along the way are diverse and challenging and in this discussion paper we shall reflect on the history of fibre optic sensors and from this endeavour to identify the characteristics of the principal stages in the process of applying concepts. Whilst this does require a somewhat different philosophy from that required for pure research, pursuing these steps is something many will find intriguing, challenging and very rewarding. © Anita Publications. All rights reserved.
Keywords: Fibre optic sensors, Product realisation, Career prospects
References
1. Udd E, Scheel E U, Fiber optic sensors: technical trends from the mid 1970’s to the present, Proc SPIE 10654, Fiber Optic Sensors and Applications XV, 106540G (14 May 2018); doi: 10.1117/12.2305531.
2. Willsch R, Kersten R T, (eds), Fiber Optic Sensors, SPIE Milestone Series. Bellingham, WA: SPIE, 1995, vol. MS108.
3. Matias Ignacio R, Villar Ignacio del (eds), Optical Fibre Sensors, to be published IEEE Sensors Press Series early 2020.
4. Martinek R, Kahankova R, Fajkus M, Nedoma J, Novak M, Jargus J, Advanced methods for fiber-optic sensor signal processing, Proc SPIE 10654, Fiber Optic Sensors and Applications XV, 106541D (14 May 2018); doi.org/10.1117/12.2304545.
5. Hartog A H, ‘An Introduction to Distributed Optical Fibre Sensors’, a volume in the CRC Series on Fibre Optic Sensors, series editor: Alexis Mendez, (CRC Press), 2017.
6. Cranch G A, Nash P J, Kirkendall C K, Large-scale remotely interrogated arrays of fiber-optic interferometric sensors for underwater acoustic applications, IEEE Sensors Journal, 3(2003)19-30 .
7. Xiao F, Chen G S, Hulsey J Leroy, Monitoring Bridge Dynamic Responses Using Fiber Bragg Grating Tiltmeters, Sensors, 17(2017)2390; doi.10.3390/s17102390,
8. Al-Fakik E, Osman N A A, Aikan F R M, The use of Fiber Bragg Garting Sensors in Biomechanics and Rehabitation Applications, Sensors, 12(2012)12890-12926; doi:10.3390/s121012890
9. LeFevre H C, The fiber optic gyroscope, 2nd Edn, (Artec House, Boston and London), 2014, p 391.
10. Lloyd S W, Dangui V, Digonnet M J F, Fan S, Kino G S, Measurement of reduced backscattering noise in laser-driven fiber optic gyroscopes, Opt Lett, 35(2010)121-123; doi.org/10.1364/OL.35.000121
11. For more on the SOFO sensor principles, history over >20 years and applications see https://smartec.ch/en/product/sofo-standard-deformation-sensor/
12. Piper I, Barnes A, Smith D, Dunn L,’ The Camino intracranial pressure sensor: is it optimal technology? An internal audit with a review of current intracranial pressure monitoring technologies’, Neurosurgery, 49(2001)1158-11164.
13. Bohnert K, Frank A, Müller G M, Yang L, Lenner M, Gabus P, Gu X, Marchese S V, Fiber optic current and voltage sensors for electric power transmission systems, Proc SPIE 10654, Fiber Optic Sensors and Applications XV, 1065402 (14 May 2018); doi: 10.1117/12.2303945;
14. Porter M E, How Competitive Forces Shape Strategy, Harvard Business Review, 59(1979)137-145.
15. Amber D, Pitch Perfect, Nature, 571(2019)589-591.
16. Gehring T, Hoff U B, Iskhakov T, Andersen U L, Towards an integrated squeezed light source, Proc. SPIE 10249, Integrated Photonics: Materials, Devices, and Applications IV, 102490D (30 May 2017); doi: 10.1117/12.2268878;
17. Budinski V, Donlagic D, Fiber-Optic Sensors for Measurements of Torsion, Twist and Rotation: A Review, Sensors, 17(2017)443; doi.org/10.3390/s17030443
18. Carotenuto B, Micco A, Ricciardi A, Amorizzo E, Mercieri M, Cutolo A, Cusano A, Optical guidance systems for epidural space identification, IEEE J Sel Top Q Electron, 23(2017)1-9.
19. Stern B, Ji X, Okawachi Y, Gaeta A L, Lipson M, Battery-operated integrated frequency comb generator, Nature, 562(2018)401-405.
20. Jin W, Ho H L, Cao Y C, Ju J, Qi L F, Gas detection with micro- and nano-engineered optical fibers, Optical Fiber Technol, 19(2013)741-759; doi.org/10.1016 /j.yofte.2013.08.004
21. Metzger N K, Spesyvtsev R, Bruce G D, Miller B, Maker G T, Malcolm G, Michael Mazilu M, Dholakia K Harnessing speckle for a sub-femtometre resolved broadband wave meter and laser stabilization. Nat Commun, 8(2017) 15610; doi: 10.1038/ncomms15610.
22. Qi Y, Zhai Y, Bao H, Jin W, Ho H L, Luo H, Nano-taper enhanced stimulated Raman gain spectroscopy for high sensitivity hydrogen detection, Proc OFS 26, paper FA3, Lausanne September, 2018 (OSA); doi.org/10.1364/OFS.2018.FA3
23. Ribeiro R S R, Dahal P, Guerreiro A, Jorge P A S,Viegas J, Fabrication of Fresnel plates on optical fibers by FIB milling for optical trapping, manipulation and detection of single cells, Sci Rep, 7(2017) 4485; doi.org/10.1038/s41598-017-04490-2
24. Zhaoa Y, Li X, Zhou X, Zhang Y, Review on the graphene based optical fiber chemical and biological sensors, Sensors and Actuators B: Chemical, 231(2016)324-340.
25. Shalaev V M, Cai W, Optical metamaterials, fundamentals and applications, (Springer), 2009
26. For the US National Photonics Initiative – https://www.lightourfuture.org/home/; for the US National Academies of Engineering – https://www.nae.edu/; and for Photonics21 Europe – https://www.photonics21.org/ (Both organisations produce regular interesting and enlightening open access reports) Meanwhile, https://photonicsscotland.com/
27. Mendez A, Krohn D A, MacDougall T W, Fiber Optic Sensors: Fundamentals and Applications, Fourth Edition (SPIE Press), 2016
Asian Journal of Physics |
Vol. 28 Nos 7-9 (2019) 567-576 |
Fibre optic chemical sensors for environmental monitoring
using sensitive nano-material coatings
Kasun Prabuddha Dissanayake1, Papiya Dhara1,2, Rahul Kumar1, Souvik Ghosh1, Hien T Nguyen1, Tong Sun1, and Kenneth T V Grattan1
1Department of Electrical and Electronic Engineering, City, University of London, London, EC1V 0HB, United Kingdom
2Department of Physics, Adamas University, Kolkata, India, 700126
Invited Article dedicated to Prof Ajoy Ghatak on his 80th Birthday
In this work the development of two different optical fibre-based chemical sensors (OFCSs), applied to better environmental monitoring, is discussed and results on the design and performance of these sensors are presented. Firstly, a graphene oxide (GO)-coated Long Period Grating (LPG)-based device, sensitive to the refractive index (RI) change caused by ambient humidity levels over the important range of 60% RH to 95% RH used for environmental monitoring, is discussed. Secondly, an OFCS device has been developed for the detection of Pb2+ ion. It was achieved by dip coating ~ 50 nm gold nanoparticles (Au-NP) on a multi-mode optical fibre and subsequently functionalizing with 1-Mercaptoundecanoic acid, to make it selective to Pb2+ ions. The Pb2+ ion sensor developed shows a sensitivity of 2.8 ×10–4 nm/µM and response time of 30s. The results obtained from these two important chemical sensor schemes highlight the importance of the use of various nanomaterials in creating novel OFCS, allowing tailoring of their performance to several environmental monitoring applications. The chemical synthesis and the functionalization of coatings such as GO and Au-NP on optical fibre paves the ways for novel OFCS device that could have important commercial potential for a wide range of applications. © Anita Publications. All rights reserved.
Keywords: Optical fibre, Plasmonics, Long-Period Grating, Graphene Oxide, Gold Nanoparticles
References
1. https://www.verifiedmarketresearch.com/product/global-sensor-market-size-and-forecast-to-2025/
2. Ziegler S, Woodward R C, Iu H H C, Borle L J, Current sensing techniques: A review, IEEE Sens J, 9(2009)354-376.
3. Ghallab Yehya H, Ismail Y, CMOS based lab-on-a-chip: Applications, challenges and future trends, IEEE Circuits Syst Mag, 14(2014)27-47.
4. Grattan K T V, Augousti A T (eds), Sensors: Technology, Systems, and Applications, (Adam Hilger, London),1991
5. Ghatak A, Thyagarajan K, Optical Electronics, (Cambridge University Press, London), 1989.
6. Ghatak A, Thyagarajan K, An Introduction to Fibre Optics, (Cambridge University Press),1998.
7. Grattan KT V, Sun T, 2000. Fibre optic sensor technology: an overview. Sens Actuators A: Phys, 82(2000)40-61.
8. Cubillas A M, Unterkofler S, Euser T G, Etzold B J, Jones A C, Sadler P J, Wasserscheid P, Russell P S J, Photonic crystal fibres for chemical sensing and photochemistry, Chem Soc Rev, 42(2013)8629-8648.
9. https://www.openpr.com/news/613292/Optical-Sensing-Market-Global-Trends-Size-Share-and-Growth-by-Forecast-to-2023.html
10. Cusano A, Paladino D, Agostino Iadicicco A, Microstructured fibre Bragg gratings, J Lightwave Technol, 27(2009)1663-1697.
11. Anna D, Blue R, Uttamchandani D, Comparative study of microfibre and side-polished optical fibre sensors for refractometry in microfluidics, IEEE Sensors Journal, 13(2013)1594-1601.
12. Chen C H, Tsao T C, Tang J L, Wu WT, 2010. A multi-D-shaped optical fibre for refractive index sensing. Sensors, 10(2010)4794-4804.
13. Luan N, Wang R, Lv W, Yao J, Surface plasmon resonance sensor based on D-shaped microstructured optical fibre with hollow core. Opt Express, 23(2015)8576-8582.
14. Cao J, Sun T, Grattan K T V, Gold nanorod-based localised surface plasmon resonance biosensors: A review, Sensors and actuators B: Chemical, 195(2014)332-351.
15. Jorgenson R C,Yee S S, 1993. A fibre-optic chemical sensor based on surface plasmon resonance. Sensors and Actuators B: Chemical, 12(1993)213-220.
16. Sharma A K, Jha R, Gupta B D, Fibre-optic sensors based on surface plasmon resonance: a comprehensive review, IEEE Sensors Journal, 7(2007)1118-1129.
17. Homola J, Yee S S, Gauglitz G, 1999. Surface plasmon resonance sensors. Sensors and Actuators B: Chemical, 54(1999)3-15.
18. Pathak A K, Ghosh S, Gangwar R K, Rahman B M A, Singh V K, Metal Nanowire Assisted Hollow Core Fiber Sensor for an Efficient Detection of Small Refractive Index Change of Measurand Liquid, Plasmonics, (2019)1-8; doi.org/10.1007/s11468-019-00969-y
19. Monzn-Hernndez David, Villatoro Joel, High-resolution refractive index sensing by means of a multiple-peak surface plasmon resonance optical fiber sensor, Sensors and Actuators B: Chemical, 115(2006)227-231.
20. Zhao Y, Li X, Zhou X, Zhang Y N, Review on the graphene based optical fibre chemical and biological sensors. Sensors and Actuators B: Chemical, 231(2016)324-340.
21. Liu C, Cai Q, Xu B, Zhu W, Zhang L, Zhao J, Chen X, Graphene oxide functionalised long period grating for ultrasensitive label-free immunosensing, Biosensors and Bioelectronics, 94(2017)200-206.
22. Hernaez M, Zamarreño C, Melendi-Espina S, Bird L, Mayes A, Arregui F, Optical fibre sensors using graphene-based materials: A review, Sensors, 17(2017)155; doi.org/10.3390/s17010155
23. Alwis L, Sun T, Grattan K V, 2012. Analysis of polyimide-coated optical fiber long-period grating-based relative humidity sensor, IEEE Sensors Journal, 13(2012)767-771.
24. Alwis L, Sun T, Grattan K T V, Design and performance evaluation of polyvinyl alcohol/polyimide coated optical fibre grating-based humidity sensors, Review of Scientific Instruments, 84(2013)025002; doi.org/10.1063/1.4789768
25. Novoselov K S, Geim A K, Morozov S V, Jiang D, Zhang Y, Dubonos S V, Grigorieva I V, Firsov A A,Electric Field Effect in Atomically Thin Carbon Films, Science, 306(2004) 666-669.
26. Yeo T L, Sun T, Grattan K T V, 2008. Fibre-optic sensor technologies for humidity and moisture measurement, Sensors and Actuators A: Physical, 144(2008)280-295.
27. Dissanayake K Prabuddha Wasantha, Vidakovic Miodrag, Matthias Fabian, Sun Tong, Kenneth T V Grattan, Graphene oxide coated Long Period Grating for Optical Sensing Purposes, J Phys, Conference Series, 1151(2019)012022; doi. 10.1088/1742-6596/1151/1/012022. .
28. Dissanayake K P W, Wu W, Nguyen H, Sun T, Grattan K T V, 2017. Graphene-oxide-coated long-period grating-based fibre optic sensor for relative humidity and external refractive index. J Lightwave Technol, 36(2017)1145-1151.
29. Nawi N M, Abdullah S, Bakar A A A, 2014, September. Gold nanoparticles/graphene oxide/polyaniline nanocomposites film as sensitive LSPR-based sensor for Pb (II) ions detection. In 2014 IEEE 5th International Conference on Photonics (ICP) (pp. 188-190). IEEE; dot.10.1109/ICP.2014.7002351 .
30. Misra N, Kumar V, Goel N K, Varshney L, Radiation synthesized poly (n-vinyl-2-pyrrolidone)-stabilized-gold nanoparticles as LSPR-based optical sensor for mercury ions estimation, J Nanopart Res, 17(2015)279; doi.org/10.1007.
31. United States Environmental Protection Agency: Children’s Health Month: EPA Offers nearly $30 million to Support Cleaner Water and Air for America’s Schoolchildren, October, 2018. Available as:https://www.epa.gov/newsreleases/childrens-health-month-epa-offers-nearly-30-million-support-cleaner-water- and-air
32. McFarland A D, Haynes C L, Mirkin C A, Van Duyne R P, Godwin H A, Color my nanoworld, J Chem Educ, 81(2004)544A; doi.org/10.1021/ed081.
33. Dhara P, Kumar R, Binetti L, Nguyen H T, Alwis L S, Sun T, Grattan K T V, Optical fiber-based heavy metal detection using the Localized Surface Plasmon Resonance technique, IEEE Sensors J, 19(2019)8720-8726.
34. Bhavsar K, Hurston E, Prabhu R, Joseph G P, Fibre optic sensor to detect heavy metal pollutants in water environments, OCEANS 2017, (pp 1-4); doi. 10.1109/OCEANSE.2017.8084982
Asian Journal of Physics |
Vol. 28 Nos 7-9 (2019) 579-585 |
Tapered thin core fiber based highly sensitive twist sensor without temperature crosstalk
Wenjun Ni1,2, Baocheng Li1,2, Chenlu Wang1,2, Yu Zheng1,2, Ran Xia1,2, Yiyang Luo1,2, Chunyong Yang3 and Perry Ping Shum2
1School of Electrical and Electronic Engineering,Nanyang Technological University, 639798, Singapore
2CINTRA CNRS/NTU/THALES, UMI 3288, Research Techno Plaza, 50 Nanyang Drive, Nanyang Technological University,637553, Singapore
3Hubei Key Laboratory of Intelligent Wireless Communications, College of Electronics and Information Engineering,
South-Central University for Nationalities, Wuhan, 430074, China
Invited Article dedicated to Prof Ajoy Ghatak on his 80th Birthday
A highly sensitive twist sensor without temperature cross sensitivity based on tapered thin core fiber (TTCF) is proposed and experimentally demonstrated. The transmission spectrum is characterized by the several dominant dips induced by the mode field mismatch between the TTCF and single mode fiber (SMF). The twist sensitivity of 0.12dB/° is achieved by tracking the intensity variation of the resonant wavelength, and the corresponding wavelength shift is located in the range of ±5pm. Similarly, the temperature sensitivity of 116pm/°C can be obtained by doing the FFT filtering on the transmission spectrum, and the corresponding power fluctuation of the selected resonant wavelength is ± 0.0006dB. Therefore, the proposed fiber sensor can realize the simultaneous measurement of twist and temperature without cross sensitivity. All these findings pave access to the practical applications of torsion measurement in the field of structural health monitoring. © Anita Publications. All rights reserved.
Keywords: Twist, Temperature, Tapered thin core fiber, Without cross sensitivity
References
1. Budinski V, Donlagic D, Fiber-Optic Sensors for Measurements of Torsion, Twist and Rotation: A Review. Sensors, 17(2017)443; doi.org/10.3390/s17030443.
2. Budinski V, Donlagic D, Quasi-distributed twist/torsion sensor, 24(2016)26282-26299.
3. Materazzi A L, Ubertini F, Eigenproperties of suspension bridges with damage, J Sound Vibrat, 330(2011)6420-6434.
4. Kim H M, Kim T H, Kim B, Chung Y, Temperature-insensitive torsion sensor with enhanced sensitivity by use of a highly birefringent photonic crystal fiber, IEEE Photon Technol Lett, 22(2010)1539-1541.
5. Wang Y P, Rao Y J, Long period fibre grating torsion sensor measuring twist rate and determining twist direction simultaneously, Electron Lett, 40(2004)164-166.
6. Chen W, Lou S, Wang L, Highly sensitive torsion sensor based on Sagnac interferometer using side-leakage photonic crystal fiber, IEEE Photon Technol Lett,
23(2011)1639-1641.
7. Wang W, Wang M, Huang X, In fiber Bragg grating twist sensor based on analysis of polarization dependent loss, Opt Express, 21(2013)11913-1920.
8. Shen C Y, Zhang Y, Zhou W J, Albert J, Au-coated tilted fiber Bragg grating twist sensor based onsurfaceplasmon resonance, Appl Phys Lett,
107(2014)071106; doi.org/10.1063/1.4865932
9. Guo T, Liu F, Guan B O, Albert J, Polarimetric multi-mode tilted fiber grating sensors, Opt Express, 22(2014)7330-7336.
10. Wang Y P, Huang X Q, Wang M, Temperature- and strain-independent torsion sensor utilizingpolarisation-dependent dependent loss of Hi-Bi FBGs, IEEE
Electron Lett, 49(2013)840-841.
11. Lesnik D, Donlagic D, In-line, fiber-optic polarimetric twist/torsion sensor, Opt Lett, 38(2013)1494-1496.
12. Budinski V and D. Donlagic, Miniature, all-fiber rotation sensor based on temperature compensated waveplate, IEEE Photon Technol Lett, 27(2015)85-88.
13. Chen Y, Semenova Y, Farrell G, Xu F, Lu Y, A Compact Sagnac Loop Based on a Microfiber Coupler for Twist Sensing, IEEE Photon Technol Lett,
27(2015)2579-2582.
14. Sun Q, Luo H, Luo H, Lai M, Liu D, Zhang L, Multimode microfiber interferometer for dual-parameters sensing assisted by Fresnel reflection, Opt Express,
23(2015)12777-12783.
15. Wang S, Lu P, Mao L, Liu D, Jiang S, Cascaded interferometers structure based on dual-pass Mach–Zehnder interferometer and Sagnac interferometer for
dual-parameter sensing, Opt Express, 23(2015)674-680.
16. Lin C, Wang Y, Huang Y, Liao C, Bai Z, Hou M, Li Z, Wang Y, Liquid modified photonic crystal fiber for simultaneous temperature and strain measurement,
Photonics Res, 5(2017)129-133.
17. Ni W, Lu P, Fu X, Sun H, Shum P P, Liao H, Jiang X, Liu D, Yang C, Zhang J, Lian Z, Simultaneous implementation of enhanced resolution and large
dynamic range for fiber temperature sensing based on different optical transmission mechanisms, Opt express, 26(2018)18341-18350.
18. Wang Y, Huang X, Wang M, Temperature Insensitive Birefringent LPG Twist Sensing Based on the Polarization Properties, IEEE Photon Technol Lett,
27(2015)2367-2370.
19. Shi L L, Zhu T, Fan Y E, Chiang K S, Rao Y J, Torsion sensing with a fiber ring laser incorporating apair of rotary long-period fiber gratings, Opt Commun,
284(2011)5299–5302.
20. Yu D, Mo Q, Hong Z, Fu S, Sima C, Tang M, Liu D, Temperature-insensitive fiber twist sensor based on elliptical-core few-mode fiber, Opt Lett,
41(2016)4617-4620.
21. Song B, Miao Y, Lin W, Zhang H, Wu J, Liu B, Multi-mode interferometer-based twist sensor with low temperature sensitivity employing square coreless
fibers, Opt Express, 21(2013)26806-26811.
22. Chen L, Zhang W, Wang L, Zhang H, Sieg J, Zhou Q, Zhang L, Wang B, Yan T, In-fiber torsion sensor based on dual polarized Mach-Zehnder interference,
Opt Express, 22(2014)31654-31664.
23. Song B, Zhang H, Xiao Y, Li W, Wu J, Liu H, Yan D, Liu B, Highly sensitive twist sensor employing Sagnac interferometer based on PM-elliptical core
fibers, Opt Express, 23(2015)15372-15379.
24. Dong X, Tam H, Shum P, Temperature -insensitive strain sensor with polarization-maintaining photonic crystal fiber based Sagnac interferometer, Appl Phys
Lett, 90(2007)151113; doi.org/10.1063/1.2722058
Asian Journal of Physics |
Vol. 28 Nos 7-9 (2019) 589-602 |
Cavity ring-down sensing techniques – A review
S O Silvaa, M B Marquesa,b, J L Santosa,b, P A S Jorgea,b and O Frazãoa,b
aINESC TEC- Institute for Systems and Computer Engineering, Technology and Science,
Rua do Campo Alegre 687, 4169-007 Porto, Portugal
bDepartment of Physics and Astronomy, Faculty of Sciences of University of Porto,
Rua do Campo Alegre 687, 4169-007 Porto, Portugal
Invited Article dedicated to Prof Ajoy Ghatak on his 80th Birthday
A review on fiber cavity ring-down (CRD) technique is presented. It addresses the main developments in this area, namely, researches involving the conventional fiber CRD configuration, i.e., cavities using two couplers with high splitting ratios, as well as the linear cavity CRD setup. Improvements of the CRD technology are discussed, where the inclusion of optical circulators and optical time domain reflectometers has been successfully demonstrated for sensing. The presented review is divided in different topics related to the measurement of physical parameters, such as strain and temperature, curvature, pressure, refractive index, gas and biochemical sensing. In particular, the work developed by our research group on this subject will be fully addressed. © Anita Publications. All rights reserved.
Keywords: Cavity-ring down, Optical fiber sensors, Physical parameters.
References
1. Berden G, Peeters R, Meijer G, Cavity ring-down spectroscopy: Experimental schemes and applications, Int Rev Phys Chem, 19(2000)565-607.
2. Zhang Y, Jin W, Yu H B, Zhang M, Liao Y B, Ho H L, Demokan M S, Stewart G, Culshaw B, Li Y H, Novel intracavity sensing network based on mode-
locked fiber laser, IEEE Photon Technol Lett, 14(2002)1336-1338.
3. Herbelin J M, McKay J A, Kwok M A, Ueunten R H, Urevig D S, Spencer D J, Benard D J., Sensitive measurement of photon lifetime and true reflectances in
an optical cavity by a phase-shift method, Appl Opt, 19(1980)144-147.
4. Anderson D Z, Frisch J C, Masser C S, Mirror reflectometer based on optical cavity decay time, Appl Opt, 23(1984) 1238-1245.
5. Wang C, Fiber loop ringdown—a time-domain sensing technique for multi-function fiber optic sensor platforms: current status and design perspectives,
Sensors, 9(2009)7595-7621.
6. Silva S O, Magalhães R, Marques M B, Frazão O, New advances in fiber cavity ring-down technology, Opt Laser Technol, 78(2016)115-119.
7. Waechter H, Litman J, Cheung A H, Barnes J A, Loock H P, Chemical sensing using fiber cavity ring-down spectroscopy, Sensors, 10(2010)1716-1742.
8. von Lerber T, Sigrist M W, Cavity-ring-down principle for fiber-optic resonators – experimental realization of bending loss and evanescent-field sensing, Appl
Opt, 41(2002)3567-3575.
9. Bo L, Jianhua L, Guiyun K, Temperature and strain sensor based on weak LPG and fiber ring down, Microw Opt Technol Lett, 50(2008)111-114.
10. Zhou K M, Webb D J, Mou C B, Farries M, Hayes N, Bennion I, Optical fiber cavity ring down measurement of refractive index with a microchannel drilled
by femtosecond laser, IEEE Photon Technol Lett, 21(2009)1653-1655.
11. Stewart G, Atherton K, Yu H, Culshaw B, An investigation of an optical fibre amplifier loop for intro-cavity and ring-down cavity loss measurements, Meas
Sci Technol, 12(2001)843-849.
12. Tarsa W P, Brzozowski D M, Rabinowitz P, Lehmann K K, Cavity ringdown strain gauge, Opt Lett, 29 (2004)1339-1341.
13. Liu X, Wang Q, Li C, Zhao C, Zhao Y, Hu H, Jin Li J, Refractive index sensor based on fiber loop ringdown spectroscopy, Instrumentation Science &
Technology, 44(2016)241-248.
14. Ni N, Chan C C, Dong X Y, Sun J, Shum P, Cavity ring-down long period fiber grating strain sensor, Meas Science Technol, 18(2007)3135-3138.
15. Lv R, Zhao Y, Liu M-C, Determination of refractive index by fiber-loop cavity ring-down spectroscopy and a long-period fiber grating, Instrumentation
Science & Technology, 44(2016)547-557.
16. Wang C, Fiber ringdown temperature sensor, Opt Eng Lett, 44(2005)030503; doi.org/10.1117/1.1869512
17. Silva S, Passos D J, Marques M B, Frazão O, Chirped Fiber Bragg Grating Cavity Ring-down for Strain Sensing Using an OTDR, Microw Opt Technol Lett,
57(2015)1442-1444.
18. Wang C, Herath C, High-sensitivity fiber-loop ringdown refractive index sensors using single-mode fiber, Opt Lett, 35(2010)1629-1631.
19. Wang Q, Liu X, Zhao Y, Lv R, Hua H, Li J, Magnetic field sensor using the fiber loop ring-down technique and an etched fiber coated with magnetic fluid,
Opt Commun, 356(2015)628-633.
20. Wong W C, Zhou W, Chan C C, Dong X, Leong K C, Cavity ring-down refractive index sensor using photonic crystal fiber interferometer, Sens Actuat B,
161(2012)108-113.
21. Yang Y, Yang L, Zhang Z, Yang J, Wang J, Zhang L, Deng X, Zhang Z, Fiber loop ring down for static ice pressure detection, Optical Fiber Technology,
36(2017)312-316.
22. Stewart G, Atherton K, Culshaw B, Cavity-enhanced spectroscopy in fiber cavities, Opt Lett, 29(2004)442-444.
23. Silva S, Magalhães R, Pérez-Herrera R A, Lopez-Amo M, Marques M B, Frazão O, Fiber cavity ring down and gain amplification effect, Photonic Sensors,
6(2016)324-327.
24. Fabian M, Lewis E, Newe T, Lochmann S, Optical fibre cavity for ring-down experiments with low coupling losses, Meas Sci Technol, 21(2010)094034;
doi.org/10.1088/0957-0233/21/9/094034.
25. Silva S, Biswas P, Bandyopadhyay S, Jorge P A, Marques M B, Frazão O, Fiber-Optic Cavity Ring Down using an Added-Signal for Curvature Sensing, IEEE
Photon Technol Lett, 27(2015)2079-2082.
26. Wang C, Scherrer S T, Fiber ringdown pressure sensors, Opt Lett, 29(2004)352-354.
27. Wang C, Scherrer S T, Fiber loop ringdown for physical sensor development: Pressure sensor, Appl Opt, 43(2004) 6458-6464.
28. Shen T, Feng Y, Sun B, Wei X, Magnetic field sensor using the fiber loop ring-down technique and an etched fiber coated with magnetic fluid, Appl Opt,
55(2016)673-678.
29. Wang Q, Xia J, Liu X, Zhao Y, Li J, Hu H, A novel current sensor based on magnetic fluid and fiber loop cavity ring-down technology, IEEE Sensors J,
15(2015)6192-6198.
30. Wang Q, Liu X, Xia J, Zhao Y, A novel long-tail fiber current sensor based on fiber loop ring-down spectroscopy and Fabry–Perot cavity filled with magnetic
fluid, IEEE Trans Instrum Meas, 64(2015)2005-2011.
31. Brown R S, Kozin I, Tong Z, Oleschuk R D, Loock H P, Fiber-loop ring-down spectroscopy, J Chem Phys, 117(2002)10444-10447.
32. Tarsa P B, Wist A D, Rabinowitz P, Lehmanna K K, Single cell detection by cavity ring-down spectroscopy, Appl Phys Lett, 85(2004)4523-4525.
33. Pu S, Gu X, Fiber loop ring down spectroscopy with a long period grating cavity, Opt Lett, 34(2009)1774-1776.
34. Ni N, Chan C, Xia L, Shum P, Fiber cavity ring-down refractive index sensor, IEEE Photon Technol Lett, 20(2008) 1351-1353.
35. Wang Q, Liu X, Xia J, Zhao Y, A novel long-tail fiber current sensor based on fiber loop ring-down spectroscopy and Fabry–Perot cavity filled with magnetic
fluid, IEEE Trans Instrum Meas, 64(2015)2005-2011.
36. Passos D J, Silva S O, Fernandes J R A, Marques M B, Frazão O, Fiber cavity ring-down using an optical time-domain reflectometer, Photonic Sensors,
4(2014)295-299.
37. Silva S, Marques M B, Frazão O, Cavity ring-down technique for remote sensing, Microw Opt Technol Lett, 58(2016)2711-2713.
38. Yüksel K, Yilmaz A, Analysis of a novel sensor interrogation technique based on fiber cavity ringdown (CRD) loop and OTDR, Optical Fiber Technology,
43(2018)57-61.
39. Silva S, Frazão O, Ring-Down technique using fiber-based linear cavity for remote sensing, IEEE Sensors Lett, 2(2018)5000704; doi.
10.1109/LSENS.2018.2847409
40. Qiu H, Qiu Y, Chen Z, Fu B, Li G, Strain Measurement by Fiber-Loop Ring-DownSpectroscopy and Fiber Mode Converter, IEEE Sensors J,
8(2008)1180-1183.
41. Ghimire M, Wang C, Highly sensitive fiber loop ringdown strain sensor with low temperature sensitivity, Meas Sci Technol, 28(2017)105101; doi.org/10.1088
/1361-6501/aa82a3
42. Qiu H, Qiu Y, Chen Z, Fu B, Chen X, Li G, Multimode fiber loop ring down spectroscopy for pressure, Microw Opt Technol Lett, 49(2007)1698-1700.
43. Zhou K M, Webb D, Farries M, Hayes N, Zhang L, Bennion I, Biochemical sensor based on a novel all-fibre cavity ring down spectroscopy technique
incorporating a tilted fibre Bragg grating, Opt Lasers Eng, 47(2009) 1023-1027.
44. Ittiarah J V, Sidhik S, Gangopadhyay T K, Refractometry using evanescent field based fiber loop ringdown spectroscopy, Sensors and Actuators A,
223(2015)61-66.
45. Wu D, Zhao Y, Wang Q, SMF taper evanescent field-based ri sensor combined with fiber loop ring down technology, IEEE Photon Technol Lett,
27(2015)1802-1805.
46. Silva S, Frazão O, Multimode interference-based fiber sensor in a cavity ring-down system for refractive index measurement, Opt Laser Technol,
91(2017)112-115.
47. Vogler D E, Muller M G, Sigrist M W, Fiber-optic cavity sensing of hydrogen diffusion, Appl Opt, 42(2003)5413-5417.
48. Zhao Y, Bai L, Wang Q, Gas concentration sensor based on fiber loop ring-down spectroscopy, Opt Commun, 309(2013)328-332.
49. Shimizu H, Noriyasu H, Measurement of carbon dioxide concentration by fiber-loop ring-down spectroscopy for continuous remote measurement, Jpn J Appl
Phys, 53(2014)116601; doi.org/10.7567/JJAP.53.116601
50. Zhao Y, Chang J, Ni J, Wang Q, Liu T, Wang C, Wang P, Lv G, Peng G, Novel gas sensor combined active fiber loop ring-down and dual wavelengths
differential absorption method, Opt Express, 22(2014)11244-11253.
51. Yolalmaza A, Sadroudb F H, Danışmana M F, Esenturk O, Intracavity gas detection with fiber loop ring down spectroscopy, Opt Commun, 396(2017)141-145.
Asian Journal of Physics |
Vol. 28 Nos 7-9 (2019) 605-620 |
Manipulation of vector beams produced in optical fiber and an application as strain sensor
Partha Roy Chaudhuri1, Saba N Khan2 and Sudip K Chatterjee3
1Department of Physics, Indian Institute of Technology Kharagpur, Kharagpur-721, 302, India
2Department of Physics, Indian Institute of Technology Delhi, New Delhi-110 016, India
3Department of Physics, Indian Institute of Technology Kanpur, Kanpur-208 016, India
Invited Article dedicated to Prof Ajoy Ghatak on his 80th Birthday
We report here our studies on the controlled generation of the doughnut-shaped zeroth-order vector modes (ZVM) alongside the corresponding scalar linearly polarized (LP) modes with a view to exploring the synthesis of various spatial vector beams by suitable superposition of the constituent basis modes. Then we present our experimental details of the controlled generation of a linearly polarized two-lobe, first-order azimuthally asymmetric beam (F-AAB) in a few-mode fiber (FMF) for a given set of input launching conditions (described with tilt and offset) and the state of polarization (SOP) of an input Gaussian mode (TEM00). A continual switching of polarization mode structure having identical intensity profile and progressive mode toggling from F-AAB to doughnut-shaped intensity pattern is demonstrated under distinct launching state. Novel optical beams namely, decentralized elliptical Gaussian beam (DEGB) possessing homogenous spatial polarization and two-grain T-polarized beam having mutually orthogonal SOP are obtained by enhancing the contribution of the fundamental mode (HE11 or, LP01) in selectively excited F-AAB. Finally, this particular study led to the designing of a highly sensitive strain measuring device owing to the large difference in propagation constants of the participating modes (LP01 and one of the F-AABs). Each of the experimentally observed intensity/polarization distributions is theoretically mapped on a one-to-one basis considering a linear superposition of appropriately excited linearly polarized basis modes of the waveguide namely, LP11 modes having both polarizations (x and y) and parity (even and odd) toward a complete understanding of the polarization and mode propagation in the few-mode structure. © Anita Publications. All rights reserved.
Keywords: Vector beams, Optical fiber, Few-mode fiber, Spatial polarization, Switching of modes, Strain sensor
References
1. Zhan Q, Cylindrical vector beams: from mathematical concepts to applications, Adv Opt Photon, 1(2009)1-57.
2. Grier D G, A revolution in optical manipulation, Nature, 424(2003)810-816.
3. Padgett M, Courtial J, Allen L, Light′s orbital angular momentum, Phys Today, 57(2004)35-40.
4. McClelland J J, Atom-optical properties of a standing-wave light field, J Opt Soc Am B, 12(1995)1761-1768.
5. Gahagan K T, Swartzlander G A, Optical vortex trapping of particles, Opt Lett, 21(1996)827-829.
6. Helseth L E, Roles of polarization, phase and amplitude in solid immersion lens system, Opt Commun, 424(2001) 161-172.
7. Pohl D, Operation of a Ruby Laser in the Purely Transverse Electric Mode TE01, Appl Phys Lett, 20(1972)266-267.
8. Zhan Q, Leger J R, Microellipsometer with radial symmetry, Appl Opt, 41(2002)4630-4637.
9. Chen H, Hao J, Zhang B-Fu, Xu J, Ding J, Wang H T, Generation of vector beams with space-variant distribution of both polarization and phase, Opt Lett,
36(2011)3179-3181.
10. Maurer C, Jesacher A, Fürhapter S, Bernet S, Ritsch-Marte M, Tailoring of arbitrary optical vector beams, New J Phys, 9(2007)1-20.
11. Grosjean T, Courjon D, Spajer M, An all-fiber device for generating radially and other polarized light beams, Opt Commun, 203(2002)1-5.
12. Volpe G, Petrov D, Generation of cylindrical vector beams with few-mode fibers excited by Laguerre–Gaussian beams, Opt Commun, 237(2004)89-95.
13. Grosjean T, Sabac A, Courjon D, A versatile and stable device allowing the efficient generation of beams with radial, azimuthal or hybrid polarizations, Opt
Commun, 252(005)12-21.
14. Viswanathan N K, Inavalli V V G K, Generation of optical vector beams using a two-mode fiber, Opt Lett, 34(2009)1189-1191.
15. Inavalli V V G K, Viswanathan N K, Switchable vector vortex beam generation using an optical fiber, Opt Commun, 283(2010)861-864.
16. Fang Z, Yao Y, Xia K, Kang M, Ueda K, Li J, Vector mode excitation in few-mode fiber by controlling incident polarization, Opt Commun,
294(2013)177-181.
17. Philip G M, Viswanathan N K, Generation of spirally polarized propagation-invariant beam using fiber microaxicon, Opt Lett, 36(2011)3906-3908.
18. Jayasurya Y V, Inavalli V V G K, Viswanathan N K, Polarization singularities in the two-mode optical fiber output, Appl Opt, 50(2011)E131-E137.
19. Kumar V, Inavalli V V G K, Viswanathan N K, Dynamic evolution of transverse energy flow in focused asymmetric optical vector-vortex beams, Opt
Commun, 285(2012)4866-4873.
20. Vyas S, Kozawa Y, Sato S, Polarization singularities in superposition of vector beams, Opt Express, 21(2013)8972-8986.
21. Novotny L, Sanchez E J, Xie X S, Near-field optical imaging using metal tips illuminated by higher-order Hermite-Gaussian beams, Ultramicroscopy,
71(1998)21-29.
22. Fatemi F K, Beadie Guy, Rapid complex mode decomposition of vector beams by common path interferometry, Opt Express, 21(2013)32291-32305.
23. Meyrath T P, Schreck F, Hanssen J L, Chuu C S, Raizen M G, A high frequency optical trap for atoms using Hermite–Gaussian beams, Opt Express,
13(2005)2843-2851.
24. Zuniga-Segundo A, Mata-Mendez O, Interaction of S-polarized beams with infinitely conducting grooves: Enhanced fields and dips in the reflectivity, Phys
Rev B, 46(1992)536-539; doi.org/10.1103/PhysRevB.46.536
25. Olivier N, Beaurepaire E, Third-harmonic generation microscopy with focus-engineered beams: a numerical study, Opt Express, 16(2008)14703-14715.
26. Luk K, Yu P, Generation of Hermite-Gaussian beam modes by multipoles with complex source points, J Opt Soc Am B, 24(1985)1818-1820.
27. Chen Y F, Huang T M, Kao C F, Wang C L, Wang S C, Generation of Hermite–Gaussian Modes in Fiber-Coupled Laser-Diode End-Pumped Lasers, IEEE J
Quantum Elect, 33(1997)1025-1031.
28. Sasada H, Okamoto M, Transverse-mode beam splitter of a light beam and its application to quantum cryptography, Phys Rev A, 68(2003)012323;
doi.org/10.1103/PhysRevA.68.012323
29. Cronin P J, High-aperture diffraction of a scalar, off-axis Gaussian beam, J Opt Soc Am A, 17(2000)1556-1564.
30. Steinbach A, Rauner M, Cruz F C, Bergquist J C, CW second harmonic generation with elliptical Gaussian beams, Opt Commun, 123(1996)207-214.
31. Rytov S M, About transition from wave to geometrical optics, Dokl Akad Nayk, 18(1938)263-267.
32. Vladimirsky V V, About rotation of a plane of polarization in the curved light beam, Dokl Akad Nayk, 21(1941)222-225.
33. Berry M V, Quantum phase factors accompanying adiabatic changes, Proc Roy Soc Lond A, 392(1984)45-57.
34. Chiao R Y, Wu Y, Manifestations of Berry’s Topological Phase for the Photon, Phys Rev Lett, 57(1986)933-936.
35. Kwiat P G, Chiao R Y, Observation of a non-classical Berry’s phase for the photon, Phys Rev Lett, 66(1991)588-591.
36. Zel’dovich B Y, Kundikova N D, Intrafibre rotation of the plane of polarisation, Quantum Elect, 25(1995)172-174.
37. Snyder A W, Love J D, Optical Waveguide Theory, (Chapman and Hall, New York, America), 1983.
38. Okamoto K, Fundamentals of Optical Waveguides, Second Edn, (American Press, California, USA), 2003.
39. Azzam R M A, Bashara N M, Ellipsometry and Polarized light, (North-Holland Publishing Company, New York, America), 1977.
40. Beijersbergen M W, Allen L, van der Veen H R L O, Woerdman J P, Astigmatic laser mode converters and transfer of orbital angular momentum, Opt
Commun, 96(1993)123-132.
41. McGloin D, Simpson N B, Padgett M J, Transfer of orbital angular momentum from a stressed fiber-optic waveguide to a light beam, Appl Opt,
37(1998)469-472.
42. Soller H, HG-LG Mode Conversion with Stressed 3-Mode Fibers under Polarization, Open J Appl Sciences, 2(2012)224-227.
43. Kalaidji D, Spajer M, Grosjean T, All-fiber controller of radial polarization using a periodic stress, Opt Lett, 36(2011)205-207..
44. Cai Y, Lin Q, The elliptical Hermite–Gaussian beam and its propagation through paraxial systems, Opt Commun, 207(2002)139-147.
45. Cai Y, Lin Q, Decentered elliptical Hermite–Gaussian beam, J Opt Soc Am A, 20(2003)1111-1119.
46. Goldstein D H, Polarized light, Third edition, (Taylor and Francis group), 2011.
47. Eftimov T A, LP01-LP11 Intermodal Interference in Highly Birefringent Two-mode Fibres with Differential LP11 polarization-mode attenuation, J Mod Opt,
42(1995)541-564.
48. Liu F, Cao D, Guo X, Lu X, Intermodal interference of LP01 and LP11 modes in panda fibers, Chin Opt Lett, 10(2012)060602.
49. Martincek I, Pudis D, Kacik D, Schuster K, Investigation of intermodal interference of LP01 and LP11 modes in the liquid-core optical fiber for temperature
measurements, Optik, 122(2011)707-710.
50. Chen W, Lou S, Wang L, Jian S, Novel modal interferometer based on ring-core photonic crystal fiber, Chin Opt Lett, 8(2010)986-988.
51. Kniazewski P, Kozacki T, Kujawinska M, Inspection of axial stress and refractive index distribution in polarization-maintaining fiber with tomographic
methods, Opt Laser Eng, 47(2009)259-263.
Asian Journal of Physics |
Vol. 28 Nos 7-9 (2019) 623-629 |
Optical Tweezers – the invention of which got the 2018 Nobel Prize in Physics
Pradeep Kumar Gupta
Physics Department, IIT Delhi, Hauz Khas, New Delhi-110016
Invited Article dedicated to Prof Ajoy Ghatak on his 80th Birthday
The widespread applications of optical tweezers particularly in biological sciences have led to the award of one half of the Nobel Prize in Physics for the year 2018 to its inventor Arthur Ashkin. In this article, I briefly describe some aspects of the work carried out by us on the use of optical tweezers for transport, rotation/orientation and sorting of microscopic objects. Use of the optical tweezers for the measurement of visco-elastic parameters of biological objects is discussed next. The use of optical tweezers to acquire spatially resolved Raman spectra from optically trapped cells and some results obtained using these so-called Raman Tweezers are also presented. © Anita Publications. All rights reserved.
Keywords: Optical Tweezers, Laser manipulation of microscopic objects, Raman tweezers
References
1. Ashkin A, Dziedzic J M, Bjorkholm J E, Chu S, Observation of a single-beam gradient force optical trap for dielectric particles, Opt Lett, 11(1986)288-290.
2. Ashkin A, Acceleration and trapping of particles by radiation pressure, Phys Rev Lett, 24(1970)156-159.
3. Ashkin A, Optical trapping and manipulation of neutral particles using lasers, (World Scientific, NJ), 2006.
4. Little H, Brown C T A, Garces-Chavez V, Sibbett W, Dholakia K, Optical guiding of microscopic particles in femtosecond and continuous wave Bessel light
beams, Opt Express, 12(2004)2560-2565.
5. Ahlawat S, Verma R S, Dasgupta R, Gupta P K, Long distance optical guiding of colloidal particles using holographic axilens, Appl Opt, 50(2011)1933-1940.
6. Mohanty S K, Gupta P K, Transport of microscopic objects using asymmetric transverse optical gradient force, Appl Phys B, 81(2005)159-162; Apparatus and
method for transport of microscopic object(s) Patent No. US 7502107 B2.
7. Mohanty S K, Sharma M, Panicker M, Gupta P K, Controlled induction, enhancement, and guidance of neuronal growth cones by use of line optical tweezers,
Opt Lett, 30 (2005)2596-2598; See also Mohanty S K, Sharma M, Panicker M, Gupta P K, Transport of Microscopic Objects Using Line Optical Tweezers:
Manipulation of Neuronal Growth Cones, Optics and Photonics News, 16(2005)19;
8. Mohanty S K, Gupta P K, Optical micromanipulation methods for controlled rotation transportation and microinjection of biological objects. In: Berns M W,
Greulich KO (eds.) Laser manipulation of cells and tissues, (Academic Press, San Diego), 2007, pp. 563-599.
9. Friese E J, Nieminen T A, Heckenberg N, Rubinsztein-Dunlop H, Optical alignment and spinning of laser-trapped microscopic particles, Nature,
394(1998)348-350.
10. Paterson L, MacDonald M P, Arit J, Sibbett W, Bryant P E, and Dholakia K, Controlled rotation of optically trapped microscopic particles, Science,
292(2001)912-914.
11. Dasgupta R, Mohanty S K, Gupta P K, Controlled rotation of biological microscopic objects using optical line tweezers, Biotechnol Lett, 25(2003)1625-1628.
See also Dasgupta R, Mohanty S K, Gupta P K, Rotating line optical tweezers enables controlled intracellular rotation of microscopic objects, Optics and
Photonics News, 14(2003)16.
12. Mohanty S K, Dasgupta R, Gupta P K, Three-dimensional orientation of microscopic objects using combined elliptical and point optical tweezers, Appl Phys
B, 81(2005)1063-1066.
13. Dasgupta R, Ahlawat S, Verma R S, Gupta P K, Optical orientation and rotation of trapped red blood cells with Laguerre-Gaussian mode, Opt Exp,
19(2011)7680-7688.
14. Dasgupta R, Verma R S, Gupta P K, Microfluidic sorting with blinking optical traps, Opt Lett, 37(2012)1739-1741.
15. Dasgupta R, Ahlawat S, Gupta P K, Microfluidic sorting with a moving array of optical traps, Appl Opt, 51(2012)4377-4387.
16. Ahlawat S, Dasgupta R, Verma R S, Kumar N V, Gupta P K, Optical sorting in holographic trap arrays by tuning the inter-trap separation, J Opt,
14(2012)125501; doi:10.1088/2040-8978/14/12/125501(12pp).
17. Dasgupta R, Gupta P K, Optical tweezers and Cytometry, in Advanced optical flow cytometry, (ed) Tuchin V V, (Wiley – VCH Verlag & Co. KGaA,
Weinheim, Germany), 2011, 363-386.
18. Mohanty S K, Uppal A, Gupta P K, Optofluidic stretching of RBCs using single optical tweezers, J Biophotonics, 1(2008)522-525.
19. Mohanty S K, Uppal A, Gupta P K, Self-rotation of red blood cells in optical tweezers: prospects for high throughput malaria diagnosis, Biotechnol Lett,
26(2004)971-974. See also Mohanty S K, Uppal A, Gupta P K, Self-rotation of red blood cells in optical tweezers: Prospects for high throughput malaria
diagnosis, Optics and Photonics News, 15(2004)19-23.
20. Mohanty S K, Mohanty K S, Gupta P K, Dynamics of Interaction of RBC with optical tweezers, Opt Express, 13(2005)4745-4751.
21. https://youtu.be/KmLVNA2nJOQ?t=976. See also reference 3 page 135
22. Patheja P, Dasgupta R, Dube A, Ahlawat S, Verma R S, Gupta P K, The use of optical trap and microbeam to investigate the mechanical and transport
characteristics of tunneling nanotubes in tumor pheroids, J Biophotonics, 8(2015)694-704.
23. Dasgupta R, Verma R S, Ahlawat S, Uppal A, Gupta P K, Studies on erythrocytes in malaria infected blood sample with Raman optical tweezers, J Biomed
Opt, 16(2011)077009; doi.org/10.1117/1.3600011
24. Ahlawat S, Kumar N, Dasgupta R, Verma R S, Uppal A, Gupta P K, Raman spectroscopic investigations on optical trap induced deoxygenation of red blood
cells, App Phys Lett,103(2013)183704; doi.org/10.1063/1.4828706
25. Ahlawat S, Kumar N, Uppal A, Gupta P K, Visible Raman excitation laser induced power and exposure dependent effects in red blood cells, J Biophotonics,
10(2017)415-422.
26. Ahlawat S, Chowdhury A, Kumar N, Uppal A, Verma R S, Gupta P K, Polarized Raman spectroscopic investigations on hemoglobin ordering in red blood
cells, J Biomed Opt,19(2014)087002; doi.org/10.1117/1.JBO.19.8.087002
27. Ahlawat S, Chowdhury A, Uppal A, Kumar N, Gupta P K, Use of Raman optical tweezers for cell cycle analysis, Analyst, 141(2016)1339-1346
Asian Journal of Physics |
Vol. 28 Nos 7-9 (2019) 631-642 |
Low-power and high-frequency optogenetic control of spiking in
ultrafast red-shifted Chrimson-expressing neurons
Himanshu Bansal, Neha Gupta and Sukhdev Roy
Department of Physics and Computer Science,
Dayalbagh Educational Institute Agra-282005, India;
Invited Article dedicated to Prof Ajoy Ghatak on his 80th Birthday
A detailed theoretical analysis and optimization of low-power temporally precise optogenetic control of high-frequency spiking in red-shifted opsin-expressing neurons has been presented. A four-state model for the photocycle of Chrimson, f-Chrimson and vf-Chrimson has been formulated and incorporated in fast spiking Wang-Buzsaki interneuron circuit model. Theoretical simulations are in excellent agreement with reported experimental results. The analysis brings out additional interesting features. Under multiple photostimulations at high-frequency, vf-Chrimson shows minimum photocurrent plateau among all-three variants. Chrimson maintains spiking fidelity upto 20 Hz, at 0.13 mW/mm2, and results in multispiking at higher frequencies. The study reveals that under optimal photostimulation protocols, f-Chrimson and vf-Chrimson both can induce spiking upto 400 Hz. Low-latency observed in spiking of vf-Chrimson-expressing neurons indicates its suitability for temporally precise high-frequency optogenetic applications. However, lower-power control allowed in f-Chrimson-expressing neurons indicates its suitability for minimizing heating effects and deep brain stimulation. The study is useful to design new low-power and high frequency optogenetic neural spiking experiments with desired spatiotemporal resolution, to provide insights into temporal spike coding and plasticity and to design neuroprosthetic devices. © Anita Publications. All rights reserved.
Keywords: Optogenetics, Channelrhodopsins, Chrimson, vf-Chrimson, f-Chrimson, Red-shifted opsins, Neural spiking, Computational optogenetics.
References
1. Chen R, Canales A, Anikeeva P, Neural recording and modulation technologies, Nat Rev Mater, 2(2017)16093; doi.org/10.1038/natrevmats.2016.93
2. Govorunova E G, Sineshchekov O A, Li H, Spudich J L, Microbial rhodopsins: diversity, mechanisms, and optogenetic applications, Annu Rev Biochem,
86(2017)845-872.
3. Gruber A, Edri O, Gepstein L, Cardiac optogenetics: the next frontier, Ep Europace, 20(2018)1910-1918.
4. Wang W, Kim C K, Ting A Y, Molecular tools for imaging and recording neuronal activity, Nat Chem Biol, 15(2019)101-110.
5. Mattis J, Tye K M, Ferenczi E A, Ramakrishnan C, O’shea D J, Prakash R, Gunaydin L A, Hyun M, Fenno L E, Gradinaru V, Yizhar O, Principles for
applying optogenetic tools derived from direct comparative analysis of microbial opsins, Nat Methods, 9(2012)159-172.
6. Wiegert J S, Mahn M, Prigge M, Printz Y, Yizhar O, Silencing neurons: tools, applications, and experimental constraints, Neuron, 95(2017)504-529.
7. Stahlberg M A, Ramakrishnan C, Willig K I, Boyden E S, Deisseroth K, Dean C, Investigating the feasibility of channelrhodopsin variants for nanoscale
optogenetics, Neurophoton, 6(2019)015007; doi: 10.1117/1.NPh.6.1.015007
8. Marshel J H, Kim Y S, Machado T A, Quirin S, Benson B, Kadmon J, Raja C, Chibukhchyan A, Ramakrishnan C, Inoue M, Shane J C, McKnight D J,
Yoshizawa S, Kato H E, Ganguli S, Deisseroth K, Cortical layer–specific critical dynamics triggering perception, Science, 365(2019)eaaw5202;
doi.10.1126/science.aaw5202
9. Köppl C, Phase locking to high frequencies in the auditory nerve and cochlear nucleus magnocellularis of the barn owl, Tyto alba, J Neurosci,
17(1997)3312-3321.
10. Foffani G, Priori A, Egidi M, Rampini P, Tamma F, Caputo E, Moxon K A, Cerutti S, Barbieri S, 300-Hz subthalamic oscillations in Parkinson’s disease,
Brain, 126(2003)2153-2163.
11. Dan Y, Poo M M, Spike timing-dependent plasticity of neural circuits, Neuron, 44(2004)23-30.
12. Moser T, Optogenetic stimulation of the auditory pathway for research and future prosthetics, Curr Opin Neurobiol, 34(2015)29-36.
13. Pan Z H, Lu Q, Bi A, Dizhoor A M, Abrams G W, Optogenetic approaches to restoring vision, Annu Rev Vis Sci, 1(2015)185-210.
14. Lewis D A, Gonzalez-Burgos G, Pathophysiologically based treatment interventions in schizophrenia, Nat Med, 12(2006)1016-1022.
15. Behrens M M, Ali S S, Dao D N, Lucero J, Shekhtman G, Quick K L, Dugan L L, Ketamine-induced loss of phenotype of fast-spiking interneurons is
mediated by NADPH-oxidase, Science, 318(2007)1645-1647.
16. Hu H, Gan J, Jonas P, Fast-spiking, parvalbumin+ GABAergic interneurons: From cellular design to microcircuit function, Science, 345(2014)1255263;
10.1126/science.1255263
17. Kann O, The interneuron energy hypothesis: implications for brain disease, Neurobiol Dis, 90(2016)75-85.
18. Buzsaki G, Penttonen M, Nadasdy Z, Bragin A, Pattern and inhibition-dependent invasion of pyramidal cell dendrites by fast spikes in the hippocampus in
vivo, Proc Natl Acad Sci USA, 93(1996)9921-9925.
19. Mager T, de la Morena D L, Senn V, Schlotte J, Feldbauer K, Wrobel C, Jung S, Bodensiek K, Rankovic V, Browne L, Huet A, High frequency neural spiking
and auditory signaling by ultrafast red-shifted optogenetics, Nat Commun, 9(2018)1750; doi.org/10.1038/s41467-018-04146-3
20. Chuong A S, Miri M L, Busskamp V, Matthews G A, Acker L C, Sørensen A T, Young A, Klapoetke N C, Henninger M A, Kodandaramaiah S B, Ogawa M,
Noninvasive optical inhibition with a red-shifted microbial rhodopsin, Nat Neurosci, 17(2014)1123-1129.
21. Guru A, Post R J, Ho Y Y, Warden M R, Making sense of optogenetics, Int J Neuropsychoph, 18(2015)pyv079; doi.org/10.1093/ijnp/pyv079
22. Zhang F, Prigge M, Beyrière F, Tsunoda S P, Mattis J, Yizhar O, Hegemann P, Deisseroth K, Red-shifted optogenetic excitation: a tool for fast neural control
derived from Volvox carteri, Nat Neurosci, 11(2008)631-633.
23. Lin J Y, Knutsen P M, Muller A, Kleinfeld D, Tsien R Y, ReaChR: a red-shifted variant of channelrhodopsin enables deep transcranial optogenetic excitation,
Nat Neurosci, 16(2013)1499-1508.
24. Erbguth K, Prigge M, Schneider F, Hegemann P, Gottschalk A, Bimodal activation of different neuron classes with the spectrally red-shifted channelrhodopsin
chimera C1V1 in Caenorhabditis elegans, PLoS one, 7(2012)e46827; doi.org/10.1371/journal.pone.0046827
25. Klapoetke N C, Murata Y, Kim S S, Pulver S R, Birdsey-Benson A, Cho Y K, Morimoto T K, Chuong A S, Carpenter E J, Tian Z, Wang J, Independent
optical excitation of distinct neural populations, Nat Methods, 11(2014)338-346.
26. Packer A M, Russell L E, Dalgleish H W, Häusser M, Simultaneous all-optical manipulation and recording of neural circuit activity with cellular resolution in
vivo, Nat Methods, 12(2015)140-146.
27. Ronzitti E, Conti R, Zampini V, Tanese D, Foust A J, Klapoetke N, Boyden E S, Papagiakoumou E, Emiliani V, Submillisecond optogenetic control of
neuronal firing with two-photon holographic photoactivation of chronos, J Neurosci, 37(2017)10679-10689.
28. Zhang Z, Russell L E, Packer A M, Gauld O M, Häusser M, Closed-loop all-optical interrogation of neural circuits in vivo, Nat Methods, 15(2018)1037-1040.
29. Schild L C, Glauser D A, Dual color neural activation and behavior control with chrimson and CoChR in Caenorhabditis elegans, Genetics,
200(2015)1029-1034.
30. Karathanos T V, Bayer J D, Wang D, Boyle P M, Trayanova N A, Opsin spectral sensitivity determines the effectiveness of optogenetic termination of
ventricular fibrillation in the human heart: a simulation study, J Physiol 594(2016)6879-6891.
31. Sengupta A, Chaffiol A, Macé E, Caplette R, Desrosiers M, Lampič M, Forster V, Marre O, Lin J Y, Sahel J A, Picaud S, Red-shifted channelrhodopsin
stimulation restores light responses in blind mice, macaque retina, and human retina, EMBO Mol Med, 8(2016)1248-1264.
32. Chen I W, Ronzitti E, Lee B R, Daigle T L, Zeng H, Papagiakoumou E, Emiliani V, Parallel holographic illumination enables sub-millisecond two-photon
optogenetic activation in mouse visual cortex in vivo, bioRxiv, (2018)250795; doi.org/10.1101/250795
33. Cheong S K, Strazzeri J M, Williams D R, Merigan W H, All-optical recording and stimulation of retinal neurons in vivo in retinal degeneration mice, PLoS
one, 13(2018)e0194947; doi.org/10.1371/journal.
34. Jun N Y, Cardin J A, Channelrhodopsin variants engage distinct patterns of network activity, bioRxiv (2018)275644; doi.org/10.1101/275644
35. Kampasi K, English D F, Seymour J, Stark E, McKenzie S, Vöröslakos M, Buzsáki G, Wise K D, Yoon E, Dual color optogenetic control of neural
populations using low-noise, multishank optoelectrodes, Microsyst Nanoeng 4(2018)10; doi.org/10.1038/s41378-018-0009-2
36. Liu M, Sharma A K, Shaevitz J W, Leifer A M, Temporal processing and context dependency in C. elegans mechanosensation, arXiv preprint
arXiv:1803.04085(2018).
37. Nikolic K, Grossman N, Grubb M S, Burrone J, Toumazou C, Degenaar P, Photocycles of channelrhodopsin-2, Photochem Photobiol, 85(2009)400-411.
38. Boyle P M, Williams J C, Ambrosi C M, Entcheva E, Trayanova N A, A comprehensive multiscale framework for simulating optogenetics in the heart, Nat
Commun, 4(2013)2370; doi: 10.1038/ncomms3370 (2013).
39. Williams J C, Xu J, Lu Z, Klimas A, Chen X, Ambrosi C M, Cohen I S, Entcheva E, Computational optogenetics: empirically-derived voltage-and light-
sensitive channelrhodopsin-2 model, PLoS Comput Biol, 9(2013)e1003220; doi.org/10.1371/journal.pcbi.1003220
40. Jarvis S, Nikolic K, Schultz S R, Neuronal gain modulability is determined by dendritic morphology: a computational optogenetic study, PLoS Comput Biol,
14(2018)e1006027; doi.org/10.1371/journal.pcbi.1006027
41. Saran S, Gupta N, Roy S, Theoretical analysis of low-power fast optogenetic control of firing of Chronos-expressing neurons, Neurophotonics,
5(2018)025009; doi.org/10.1117/1.NPh.5.2.025009
42. Urmann D, Lorenz C, Linker S M, Braun M, Wachtveitl J, Bamann C, Photochemical Properties of the Red-shifted Channelrhodopsin Chrimson, Photochem
Photobiol, 93(2017)782-795.
43. Roy S, Singh C P, Reddy K P, Generalized model for all-optical light modulation in bacteriorhodopsin, J Appl Phys, 90(2001)3679-3688.
44. Roy S, Kikukawa T, Sharma P, Kamo N, All-optical switching in pharaonis phoborhodopsin protein molecules, IEEE Trans Nanobiosci, 5(2006)178-87.
45. Roy S, Yadav C, All-optical sub-ps switching and parallel logic gates with bacteriorhodopsin (BR) protein and BR-gold nanoparticles, Laser Phys Lett,
11(2014)125901; doi.org/10.1088/1612-2011/11/12/125901
46. Schneider F, Grimm C, Hegemann P, Biophysics of channelrhodopsin, Annu Rev Biophys, 44(2015)167-86.
47. Oda K, Vierock J, Oishi S, Rodriguez-Rozada S, Taniguchi R, Yamashita K, Wiegert J S, Nishizawa T, Hegemann P, Nureki O, Crystal structure of the red
light-activated channelrhodopsin Chrimson, Nat Commun, 9(2018)3949; doi.org/10.1038/s41467-018-06421-9
48. Evans B D, Jarvis S, Schultz S R, Nikolic K, PyRhO: a multiscale optogenetics simulation platform, Front Neuroinform, 10(2016)1-19.
49. Gupta N, Bansal H, Roy S, Theoretical optimization of high-frequency optogenetic spiking of red-shifted very fast-Chrimson-expressing neurons,
Neurophoton, 6(2019)025002; doi.org/10.1117/1.NPh.6.2.025002
50. Hodgkin A L, Huxley A F, A quantitative description of membrane current and its application to conduction and excitation in nerve, J Physiol,
117(1952)500-544.
51. Wang X J, Buzsáki G, Gamma oscillation by synaptic inhibition in a hippocampal interneuronal network model, J Neurosci, 16(1996)6402-6413.
52. Stefanescu R A, Shivakeshavan R G, Khargonekar P P, Talathi S S, Computational modeling of channelrhodopsin-2 photocurrent characteristics in relation to
neural signalling, Bull Mathemat Biol, 75(2013)2208-2240.
53. Kim C K, Adhikari A, Deisseroth K, Integration of optogenetics with complementary methodologies in systems neuroscience, Nat Rev Neurosci,
18(2017)222-235.
54. Gunaydin L A, Yizhar O, Berndt A, Sohal V S, Deisseroth K, Hegemann P, Ultrafast optogenetic control, Nat Neurosci, 13(2010)387-392;
doi:10.1038/nn.2495.
Asian Journal of Physics |
Vol. 28 Nos 7-9 (2019) 645-653 |
All-dielectric metasurface for complex-amplitude modulation for linearly polarized light
Jangwoon Sung, Gun-Yeal Lee, Jongwoo Hong, Hyunwoo Son and Byoungho Lee
Inter-University Semiconductor Research Center and School of Electrical and Computer Engineering,
Seoul National University, Gwanak-GuGwanakro 1, Seoul 08826, South Korea
Invited Article dedicated to Prof Ajoy Ghatak on his 80th Birthday
We propose a simple-shaped metasurface platform that can modulate phase and amplitude independently. The proposed structure is designed to modulate the phase and amplitude independently of the co-polarized light when the linearly polarized light is incident. By fine-tuning structural parameters of silicon nanorods, we achieved independent control of amplitude and phase at linearly polarized light incidence condition. Maximum efficiency of proposed scheme has reached up to 90%, which is verified numerically. This scheme has potential to be applied in various holographic applications, which require linearly polarized light. © Anita Publications. All rights reserved.
Keywords: Metasurfaces, Metamaterials, Complex-amplitude modulation
References
1. Chen H-T, Taylor A J, Yu N, A review of metasurfaces: physics and applications , Rep Prog Phys, 79(2016):076401; doi.org/10.1088/0034-4885/79/7/076401.
2. Glybovski S B,Tretyakov S A, Belov P A, Kivshar Y S, Simovski C R, Metasurfaces: From microwaves to visible, Phys Rep, 634(2016)1-72.
3. Genevet P, Capasso F, Aieta F, Khorasaninejad M, Devlin R, Recent advances in planar optics: from plasmonic to dielectric metasurfaces, Optica,
4(2017)139-152.
4. Hsiao H-H, Chu C H, Tsai D P, Fundamentals and Applications of Metasurfaces, Small Methods,1(2017):1600064; doi.org/10.1002/smtd.201600064
5. Kruk S, Kivshar Y, Functional meta-optics and nanophotonics governed by Mie resonances, ACS Photonics, 4(2017)2638-2649.
6. Chen M, Kim M, Wong A M H, Eleftheriades G V, Huygens’ metasurfaces from microwaves to optics: a review, Nanophotonics, 7(2018)1207;
doi.org/10.1515/nanoph-2017-0117
7. Lee G, Sung J, Lee B, ETRI Journal, Recent advances in metasurface hologram technologies, 41(2019)10-22; doi.org/10.4218/etrij.2018-0532.
8. Sung J, Lee G-Y, Lee B, Progresses in the practical metasurface for holography and lens, Nanophotonics, 8(2019)1701-1718; doi.org/10.1515/nanoph-
2019-0203
9. Chen W T, Yang K Y, Wang C M, Huang Y W, Sun G, Chiang I D, Liao C Y, Hsu W L, Lin H T, Sun S, Zhou L, Liu A Q, Tsai D P, High-efficiency
broadband meta-hologram with polarization-controlled dual images, Nano Lett, 14(2014)225-230.
10. Arbabi A, Horie Y, Bagheri M, Faraon A, Arbabi A, Horie Y, Bagheri M, Faraon A, Dielectric metasurfaces for complete control of phase and polarization
with subwavelength spatial resolution and high transmission, Nature Nanotech, 10(2015)937-943.
11. Lee G-Y, Yoon G, Lee S Y, Yun H, Cho J, Lee K, Kim H, Rho J,Lee B, Complete amplitude and phase control of light using broadband holographic
metasurfaces, Nanoscale, 10(2018)4237-4245.
12. Sung J, Lee G-Y, Choi C, Hong J, Lee B, Single-Layer Bifacial Metasurface: Full-Space Visible Light Control, Adv Opt Mater, 7(2019)1801748;
doi.org/10.1002/adom.201801748
13. Khorasaninejad M, Chen W T, Devlin R C, Oh J, Zhu A Y, Capasso F, Metalenses at visible wavelengths: Diffraction-limited focusing and subwavelength
resolution imaging, Science, 352(2016)1190-1194.
14. Chen W T, Zhu A Y, Sanjeev V, Khorasaninejad M, Shi Z, Lee E, Capasso F, A broadband achromatic metalens for focusing and imaging in the visible, Nat
Nanotech, 13(2018)220-226.
15. Lee K,Yun H, Mun S-E, Lee G-Y, Sung J, Lee B, Ultracompact Broadband Plasmonic Polarimeter, Laser & Photon Rev, 12(2018)1700297; doi.org/10.1002
/lpor.201700297
16. Lee G-Y, Hong J Y, Hwang S H, Moon S, Kang H, Jeon S, Kim H, Jeong J-H, Lee B, Metasurface eyepiece for augmented reality, Nat Commun, 9(2018)4562;
doi.org/10.1038/s41467-018-07011-5
17. Lan S, Zhang X, Taghinejad M, Rodrigues S, Lee K-T, Liu Z, Cai W, Metasurfaces for near-eye augmented reality, ACS Photonics, 6(2019)864-870.
18. Arbabi E, Li J, Hutchins R J, Kamali S M, Arbabi A, Horie Y, Dorpe P V, Gradinaru V, Wagenaar D A, Faraon A, Two-Photon Microscopy with a Double-
Wavelength Metasurface Objective Lens, Nano Lett, 18(2018)4943-4948.
19. Huang L, Chen X, Mühlenbernd H, Zhang H, Chen S, Bai B, Tan Q, Jin G, Cheah K-W, Qiu C-W, Li J, Zentgraf T, Zhang S, Three-dimensional optical
holography using a plasmonic metasurface, Nat Commun, 4(2013)2808 ; doi: 0.1038/ncomms3808
20. Zheng G, Mühlenbernd H, Kenney M, Li G, Thomas Zentgraf T, Zhang S, Metasurface holograms reaching 80% efficiency, Nat Nanotech, 10(2015)308-312.
21. Mueller J P B, Rubin N A, Devlin R C, Groever B Capasso F, Metasurface Polarization Optics: Independent Phase Control of Arbitrary Orthogonal States of
Polarization, Phys Rev Lett, 118(2017)113901; doi.org/10.1103/PhysRevLett.118.113901
22. Chen W T, Zhu A Y, Sisler J, Huang Y-W, Yousef K M A, Lee E, Qiu C-W, Capasso F, Broadband Achromatic Metasurface-Refractive Optics, Nano Lett,
18(2018)7801-7808
23. Chen W T, Zhu A Y, Sisler J, Bharwani Z, Federico Capasso F, A broadband achromatic polarization-insensitive metalens consisting of anisotropic
nanostructures, Nat Commun, 10(2019)355; doi.org/10.1038/s41467-019-08305-y
24. Yu N, Genevet P, Kats M A, Aieta F, Light Propagation with Phase Discontinuities: Generalized Laws of Reflection and Refraction, Science,
334(2011)333-337.
Asian Journal of Physics |
Vol. 28 Nos 7-9 (2019) 655-660 |
Metasurfaces for magnetic field enhancement
Keshav Samrat Modi1,2, Jasleen Kaur1,2, Satya Pratap Singh2, Umesh Tiwari1,2 and Ravindra Kumar Sinha1,2,3
1Academy of Scientific and Innovative Research (AcSIR), Ghaziabad-201 002, India
2CSIR- Central Scientific Instruments Organisation, Chandigarh, India-160 030
3TIFAC-Center of Relevance and Excellence in Fiber Optics and Optical Communication,
Delhi Technological University, Delhi-110 042, India
Invited Article dedicated to Prof Ajoy Ghatak on his 80th Birthday
In this paper, we present the comparison of different metasurfaces which shows magnetic field enhancement when interacts with RF radiation. In the presence of the electromagnetic field, the proposed metasurfaces redistribute the electromagnetic field such that magnetic field gets enhanced while electric field gets diminished over the centre of the metasurface. The enhancement in the magnetic field is required for improvement of the signal-to-noise ratio. The different metasurfaces are compared based on their characteristic response to the interacting electromagnetic field. © Anita Publications. All rights reserved.
Keywords: Metasurfaces, Resonance, Field enhancement and Metalo-dielectric structure
References
1. Prakash M, Sinha A, Dhillon M, Khandelwal N, Pictorial magnetic Resonance Imaging Findings in Common Sports Injuries of Knee, Journal of Postgraduate
Medicine, Education Research, 50(2016)151-155.
2. Patkar D P, Yanamandala R, Pardhi M, Pungavkar S A, Gaikwad V, Pawar S V, MRI of lateral skull base lesions, Otorhinolaryngology Clinics: An
International Journal, 3(2011)43-55.
3. Moreau F, Asdaghi N, Modi J, Goyal M, Coutts S B, Magnetic Resonance Imaging versus Computed Tomography in Transient Ischemic Attack and Minor
Stroke: The More Υou See the More You Know, Cerebrovasc Dis Extra, 3(2013)130-136.
4. Ljubic A, Cetkovic A, Mikic A N, Stamenkovic J D, Jovanovic I, Opincal T S, Damnjanovic D, Ultrasound vs MRI in Diagnosis of Fetal and Maternal
Complications, Donald School J Ultrasound Obstet Gynecol , 5(2011)231-242.
5. Slobozhanyuk A P, Poddubny A N, Raaijmakers A J, Berg C A van den, Kozachenko A V, Dubrovina I A, Melchakova I V, Kivshar Y S, Belov P A,
Enhancement of Magnetic Resonance Imaging with Metasurfaces, Adv Mater, 28(2016)1832-1838.
6. Ibrahim R, Mazli M, Amrizal M, Aljunid S M, Cost of magnetic resonance imaging (MRI) and computed tomography (CT) scan in UKMMC, BMC Health
Services Research, 12(2012)11; doi.org/10.1186/1472-6963-12-S1-P11
7. Schmidt R, Slobozhanyuk A, Belov P, Webb A, Flexible and compact hybrid metasurfaces for enhanced ultra high field in vivo magnetic resonance imaging,
Sci Rep, 7(2017)1678; doi.org/10.1038/s41598-017-01932-9
8. Modi K S, Singh S P, Tiwari U, Sinha R, Presented at the Symposium on “30 years of Photonic Crystals – the Indian research scenario”, Indian Institute of
Technology, Kanpur, India, 2017.
9. Modi K S, Singh S P, Tiwari U, Sinha R, Presented at the International Conference on Advances in Optics & Photonics (ICAOP 2017) Proceedings of XLI
Conference of Optical Society of India, Guru Jambheshwar University of Science & Technology, Hisar, Haryana, India, 2017.
10. Modi K S, Singh S P, Tiwari U, Sinha R, Presented at the 3rd International Conference on Microwave and Photonics (ICMAP-2018), Indian Institute of
Technology, (Indian School of Mines), Dhanbad, India, 2018.
11. Brown R W, Haacke E M, Cheng Y-C N, Thompson M R, Venkatesan R, Magnetic resonance imaging: physical principles and sequence design, (John Wiley
& Sons), 2014.
12. Gauthier N, Magnetic field of an infinite current-carrying ribbon, Am J Phys, 56(1988)819; doi.org/10.1119/1.15456
Asian Journal of Physics |
Vol. 28 Nos 7-9 (2019) 663-685 |
Fano Resonances in Terahertz Metamaterials
Subhajit Karmakar1, Ravendra K Varshney1, Dibakar Roy Chowdhury2 and Bishnu P Pal2
1Department of Physics, Indian Institute of Technology Delhi, New Delhi, 110 016, India
2Department of Physics, Mahindra Ecole Centrale, Jeedimetla, Hyderabad, 500 043, India
Invited Article dedicated to Prof Ajoy Ghatak on his 80th Birthday
Fano resonance is an important phenomenon in physics. Unlike in conventional Lorentzian resonances, normally Fano resonance is characterized by sharp, asymmetrical spectral features that are induced by interference phenomenon. During recent years Fano modes have attracted intense interest in metamaterial systems too. This review is intended to present history, physics and properties of various devices that exploit Fano resonance at terahertz frequency domain in the field of metamaterials systems. © Anita Publications. All rights reserved.
Keywords: Fano resonance, Metamaterials, Terahertz, Optical devices.
References
1. Pendry J B, Holden A J, Robbins D J, Stewart W J, Magnetism from conductors and enhanced nonlinear phenomena, IEEE Transactions on Microwave
Theory and Techniques, 47(1999)2075-2084.
2. Smith D R, Pendry J B, Wiltshire M C K, Metamaterials and Negative Refractive Index, Science, 305(2004)788-792.
3. Pendry J B, Negative Refraction Makes a Perfect Lens, Phys Rev Lett, 85(2000)3966; doi.10.1103/PhysRevLett.85.3966
4. Chowdhury D R, Xu N, Zhang W, Singh S, Resonance tuning due to Coulomb interaction in strong near-field coupled metamaterials, J Appl Phys,
118(2015)023104; doi.org/10.1063/1.4926758
5. Pendry J B, Schurig D, Smith D R, Controlling Electromagnetic Fields, Science, 312(2006)1780-1782.
6. Huang Y W, Chen W T, Wu P C, Fedotov V A, Zheludev N I, Tsai D P, Toroidal Lasing Spaser, Sci Rep, 3(2013)1237; doi.org/10.1038/srep01237
7. Ziolkowski R W, Ultrathin, metamaterial-based laser cavities, J Opt Soc Am B, 23(2006)451-460.
8. Gao W, Chen S J, Withayachumnankul W, Fumeaux C, Horizontally Polarized 360° Beam-Steerable Frequency-Reconfigurable Antenna, IEEE Transactions on Antennas and Propagation, 67(2019)5231-5242.
9. Driscoll T, Kim H T, Chae B G, Kim B J, Lee Y W, Jokerst N M, Palit S, Smith D R, Ventra M D, Basov D N, Memory Metamaterials, Science, 325(2009)1518-1521.
10. Lee H, Arakelyan, S, Friedman B, Lee K, Hybrid Resonance Memory Metamaterial with Full-Wave Operation Adv Funct Mater, 28(2018)1800760; doi: 10.1002/adfm.201800760
11. Khurgin J B, How to deal with the loss in plasmonics and metamaterials, Nat Nanotechnol, 10(2015)2-6.
12. Lagarkov A N, Kisel V N, Losses in metamaterials: Restrictions and benefits, Physica B: Condensed Matter, 405 (2010)2925-2929.
13. Husnik M, Klein M W, Feth N, König M, Niegemann J, Busch K, Linden S, Wegener M, Absolute extinction cross-section of individual magnetic split-ring resonators, Nat Photonics, 2(2008)614-617.
14. Limonov M F, Rybin M V, Poddubny A N, Kivshar Y S, Fano resonances in photonics, Nat Photonics, 11(2017) 543-554.
15. Luk’yanchuk B, Zheludev N I, Maier S A, Halas N J, Nordlander P, Giessen H, Chong C T, The Fano resonance in plasmonic nanostructures and metamaterials, Nat Mater, 9(2010)707-715.
16. Barh A, Pal B P, Agrawal G P, Varshney R K, Rahman B M A, Specialty Fibers for Terahertz Generation and Transmission: A Review, IEEE J Sel Top Quantum Electron, 22(2016)365-379.
17. Kulesa C, Terahertz Spectroscopy for Astronomy: From Comets to Cosmology, IEEE Transactions on Terahertz Science and Technology, 1(2011)232-240.
18. Yang Y, Mandehgar M, Grischkowsky D R, Understanding THz Pulse Propagation in the Atmosphere, in IEEE Transactions on Terahertz Science and Technology, 2(2012)406-415.
19. Ho L, Pepper M, Taday P, Terahertz spectroscopy: signatures and fingerprints, Nat Photonics, 2(2008)541-543.
20. Davies A G, Burnett A D, Fan W, Linfield E H, Cunningham J E, Terahertz spectroscopy of explosives and drugs, Materials Today, 11(2008)18-26.
21. Puc U, Abina A, Rutar M, Zidanšek A, Jeglič A, Valušis G, Terahertz spectroscopic identification of explosive and drug simulants concealed by various hiding techniques, Appl Opt, 54(2015)4495-4502.
22. Köhler R, Tredicucci A, Beltram F, Beere H E, Linfield E H, Davies A G, Ritchie D A, Iotti R C, Rossi F, Terahertz semiconductor-heterostructure laser, Nature, 417(2002)156-159.
23. Chassagneux Y, Colombelli R, Maineult W, Barbieri S, Beere H E, Ritchie D A, Khanna S P, Linfield E H, Davies A G, Electrically pumped photonic-crystal terahertz lasers controlled by boundary conditions, Nature, 457(2009) 174-178.
24. Miroshnichenko A E, Flach S, Kivshar Y S, Fano resonances in nanoscale structures, Rev Mod Phys, 82(2010) 2257-2298.
25. Beutler H, Über Absorptionsserien von Argon, Krypton und Xenon zu Termen zwischen den beiden Ionisierungsgrenzen 2P32/0 und 2P12/0, Z Physik, 93(1935)177-196.
26. Fano U, Sullo spettro di assorbimento dei gas nobili presso il limite dello spettro d’arco, Il Nuovo Cimento, 12(1935) 154-161.
27. Fano U, Effect of Configuration interactions on Intensities and phase shifts, Phys Rev, 124(1961)1866-1878.
28. Redner S, Citation Statistics From More Than a Century of Physical Review, arXiv:physics/0407137 (2004).
29. Wood R W, On a Remarkable Case of Uneven Distribution of Light in a Diffraction Grating Spectrum, Proc Phys Soc London, 18(1902)269; doi.org/10.1088/1478-7814/18/1/325
30. Wood R W, Anomalous Diffraction Gratings, Phys Rev, 48(1935)928; doi.org/10.1103/PhysRev.48.928
31. Strutt J W, On the dynamical theory of gratings, Proc Roy Soc London, 79(1907)399-416; doi.org/10.1098/rspa.1907.0051
32. Karmakar S, Varshney R K, Chowdhury D R, Theoretical investigation of active modulation and enhancement of Fano resonance in THz metamaterials, OSA Continuum, 2(2019)531-539.
33. Joe Y S, Satanin A M, Kim C S, Classical analogy of Fano resonances, Phys Scr, 74(2006)259-266.
34. Han S, Cong L, Lin H, Xiao B, Yang H, Singh R, Tunable electromagnetically induced transparency in coupled three-dimensional split-ring-resonator metamaterials, Sci Rep, 6(2016)20801; doi.org/10.1038/srep20801
35. Fedotov V A, Rose M, Prosvirnin S L, Papasimakis N, Zheludev N I, Sharp Trapped-Mode Resonances in Planar Metamaterials with a Broken Structural Symmetry, Phys Rev Lett, 99(2007)147401; doi.org/10.1103/PhysRevLett.99.147401
36. Zhang S, Genov D A, Wang Y, Liu M, Zhang X, Plasmon-induced transparency in metamaterials, Phys Rev Lett,101 (2008)047401; doi.org/10.1103/PhysRevLett.101.047401
37. Papasimakis N, Fedotov V A, Zheludev N I, Prosvirnin S L, Metamaterial analog of electromagnetically induced transparency, Phys Rev Lett,101(2008) 253903; doi.org/10.1103/PhysRevLett.101.253903
38. Liu N, Kaiser S, Giessen H, Magnetoinductive and Electroinductive Coupling in Plasmonic Metamaterial Molecules, Adv Mater, 20(2008)4521-4525.
39. Christ A, Martin O J F, Ekinci Y, Gippius N A, Tikhodeev S G, Symmetry breaking in a plasmonic metamaterial at optical wavelength, Nano Lett, 8(2008)2171-2175.
40. Singh R, Plum E, Menzel C, Rockstuhl C, Azad A K, Cheville R A, Lederer F, Zhang W, Zheludev N I, Terahertz metamaterial with asymmetric transmission, Phys Rev B, 80(2009)153104; doi.org/10.1103/PhysRevB.80.153104
41. Papasimakis N, Fedotov V A, Fu Y H, Tsai D P, Zheludev N I, Coherent and incoherent metamaterials and order-disorderr transitions, Phys Rev B, 80(2009)041102; doi.org/10.1103/PhysRevB.80.041102
42. Chiam S Y, Singh R, Rockstuhl C, Lederer F, Zhang W, Bettiol A A, Analogue of electromagnetically induced transparency in terahertz metamaterial, Phys Rev B, 80(2009)153103; doi.org/10.1103/PhysRevB.80.153103
43. Manjappa M, Chiam S Y, Cong L, Bettiol A A, Zhang W, Singh R, Tailoring the slow light behavior in terahertz metasurfaces, Appl Phys Lett, 106(2015)181101; doi.org/10.1063/1.4919531
44. Singh R, Al-Naib I A I, Koch M, Zhang W, Sharp Fano resonances in THz metamaterials, Opt Express, 19(2011) 6312-6319.
45. Singh R, Al-Naib I A I, Cao W, Rockstuhl C, Koch M Zhang W, The Fano Resonance in Symmetry Broken Terahertz Metamaterials, IEEE Transactions on Terahertz Science and Technology, 3(2013)820-826.
46. Pryce I M, Aydin K, Kelaita Y A, Briggs R M, Atwater H A, Highly strained compliant optical metamaterials with large frequency tunability, Nano Lett, 10(2010)4222-4227.
47. Aydin K, Pryce I M, Atwater H A, Symmetry breaking and strong coupling in planar optical metamaterials, Opt Express, 18(2010)13407-13417.
48. Lukyanchuk B, Zheludev N I, Maier S A, Halas N J, Nordlander P, Giessen H, Chong C T, The Fano resonance in plasmonic nanostructures and metamaterials, Nat Mater, 9(2010)707-715.
49. Mukherjee S, Sobhani H, Lassiter J B, Bardhan R, Nordlanderv P, Halas N J, Fanoshells: Nanoparticles with Built-in Fano Resonances, Nano Lett, 10(2010)2694-2701.
50. Fan J A, Bao K, Wu C, Bao J, Bardhan R, Halas N J, Manoharan V N, Shvets G, Nordlander P, Capasso F, Fano-like Interference in Self-Assembled Plasmonic Quadrumer Clusters, Nano Lett, 10(2010)4680-4685.
51. González P G, Albella P, Golmar F, Arzubiaga L, Casanova F, Hueso L E, Aizpurua J, Hillenbrand R, Visualizing the near-field coupling and interference of bonding and anti-bonding modes in infrared dimer nanoantennas, Opt Express, 21(2013)1270-1280.
52. Chuntonov L, Haran G, Trimeric Plasmonic Molecules: The Role of Symmetry, Nano Lett, 11(2011)2440-2445.
53. Dregely D, Hentschel M, Giessen H, Excitation and Tuning of Higher-Order Fano Resonances in Plasmonic Oligomer Clusters ACS Nano, 5(2011)8202-8211.
54. Lassiter J B, Sobhani H, Knight M W, Mielczarek W S, Nordlander P, Halas N J, Designing and Deconstructing the Fano Lineshape in Plasmonic Nanoclusters, Nano Letts, 12(2012)1058-1062.
55. Francescato Y, Giannini V, Maier S A, Plasmonic Systems Unveiled by Fano Resonances, ACS Nano, 6(2012) 1830-1838.
56. Zhang Q, Wen X, Li G, Ruan Q, Wang J, Xiong Q, Multiple Magnetic Mode-Based Fano Resonance in Split-Ring Resonator/Disk Nanocavities, ACS Nano, 7(2013)11071-11078.
57. Dong Z G, Liu H, Xu M X, Li T, Wang S M, Zhu S N, Zhang X, Plasmonically induced transparent magnetic resonance in a metallic metamaterial composed of asymmetric double bars, Opt Express, 18(2010)18229-18234.
58. Artar A, Yanik A A, Altug H, Directional Double Fano Resonances in Plasmonic Hetero-Oligomers, Nano Lett, 11(2011)3694-3700.
59. Lovera A, Gallinet B, Nordlander P, Martin O J F, Mechanisms of Fano Resonances in Coupled Plasmonic Systems, ACS Nano, 7(2013)4527-4536.
60. Mun S E, Yun H, Choi C, Kim S J, Lee B, Enhancement and switching of fano resonance in metamaterial, Adv Opt Mater, 6(2018)1800545; doi.org/10.1002/adom.201800545
61. Moritake Y, Kanamori Y, Hane K, Demonstration of sharp multiple Fano resonances in optical metamaterials, Opt Express, 24(2016)9332-9339.
62. Yang Z J, Zhang Z S, Zhang L H, Li Q Q, Hao Z H, Wang Q Q, Fano resonances in dipole-quadrupole plasmon coupling nanorod dimers, Opt Lett, 36(2011)1542-1544.
63. Singh R, Al-Naib I A I, Yang Y, Chowdhury D R, Cao W, Rockstuhl C, Ozaki T, Morandotti R, Zhang W, Observing metamaterial induced transparency in individual Fano resonators with broken symmetry, Appl Phys Lett, 99(2011) 201107; doi.org/10.1063/1.3659494
64. Ma Y, Li Z, Yang Y, Huang R, Singh R, Zhang S, Gu J, Tian Z, Han J, Zhang W, Plasmon-induced transparency in twisted Fano terahertz metamaterials, Opt Mater Express, 1(2011)391-399.
65. Li Z, Ma Y, Huang R, Singh R, Gu J, Tian Z, Han J, Zhang W, Manipulating the plasmon-induced transparency in terahertz metamaterials, Opt Express, 19(2011)8912-8919.
66. Giannini V, Francescato Y, Amrania H, Phillips C C, Maier S A, Fano Resonances in Nanoscale Plasmonic Systems: A Parameter-Free Modeling Approach, Nano Letts, 11(2011)2835-2840.
67. Plum E, Tanaka K, Chen W T, Fedotov V A, Tsai D P, Zheludev N I, A combinatorial approach to metamaterials discovery, J Opt, 13(2011)055102; doi.org/10.1088/2040-8978/13/5/055102
68. Wood R W, On a remarkable case of uneven distribution of light in a diffraction grating spectrum, The London, Edinburgh, and Dublin Philosophical Magazine and Journal of Science, 4(1902)396-402.
69. Tan T C, Srivastava Y K, Manjappa M, Plum E, Singh R, Lattice induced strong coupling and line narrowing of split resonances in metamaterials, Appl Phys Lett, 112(2018)201111; doi.org/10.1063/1.5026649
70. Verslegers L, Yu Z, Ruan Z, Catrysse P B, Fan S, From electromagnetically induced transparency to superscattering with a single structure: a coupled-mode theory for doubly resonant structures, Phys Rev Lett, 108(2012)083902; doi.org/10.1103/PhysRevLett.108.083902
71. Liu S D, Yang Z, Liu R P, Li X Y, Multiple Fano Resonances in Plasmonic Heptamer Clusters Composed of Split Nanorings, ACS Nano, 6(2012)6260-6271.
72. Gupta M, Singh R, Toroidal versus Fano resonances in high-Q planar THz metamaterials, Adv Opt Mater, 4(2016) 2119-2125.
73. Cong L, Manjappa M, Xu N, Al-Naib I A I, Singh R, Fano resonances in terahertz metasurfaces: a figure of merit optimization, Adv Opt Mater, 3(2015)1537-1543.
74. Cao W, Singh R, Al-Naib I A I, He M, Taylor A J, Zhang W, Low-loss ultra-high-Q dark mode plasmonic Fano metamaterials, Opt Lett, 37(2012)3366-3368.
75. Lim W X, Singh R, Universal behaviour of high-Q Fano resonances in metamaterials: terahertz to near-infrared regime, Nano Converg, 5(2018); doi.org/10.1186/s40580-018-0137-2.
76. Kim J, Soref R, Buchwald W R, Multi-peak electromagnetically induced transparency (EIT)-like transmission from bull’s-eye-shaped metamaterial, Opt Express, 18(2010)17997-18002.
77. Wu C, Khanikaev A B, Shvets G, Broadband Slow Light Metamaterial Based on a Double-Continuum Fano Resonance, Phys Rev Lett, 106(2011)107403; doi.org/10.1103/PhysRevLett.106.107403
78. Zhu L, Meng F Y, Fu J H, Wu Q, Hua J, Multi-band slow light metamaterial, Opt Express, 20(2012)4494-4502.
79. Wang J, Fan C, He J, Ding P, Liang E, Xue Q, Double Fano resonances due to interplay of electric and magnetic plasmon modes in planar plasmonic structure with high sensing sensitivity, Opt Express, 21(2013)2236-2244.
80. Born N, Al-Naib I A I, Jansen C, Ozaki T, Morandotti R, Koch M, Excitation of multiple trapped-eigenmodes in terahertz metamolecule lattices, Appl Phys Lett, 104(2014)101107; doi.org/10.1063/1.4868420
81. Yang S, Tang C, Liu Z, Wang B, Wang C, Li J, Wang L, Gu C, Simultaneous excitation of extremely high-Q-factor trapped and octupolar modes in terahertz metamaterials, Opt Express, 25(2017)15938-15946.
82. Cao T, Wei C, Simpson R E, Zhang L, Cryan M J, Fast tuning of double Fano resonance using a phase-change metamaterial under low power intensity, Sci Rep, 4(2014)4463; doi.org/10.1038/srep04463
83. Zhang Y, Li T, Zeng B, Zhang H, Lv H, Huang X, Zhang W, Azad A K, A graphene based tunable terahertz sensor with double Fano resonances, Nanoscale, 7(2015)12682-12688.
84. Devi K M, Islam M, Chowdhury D R, Sarma A K, Kumar G, Plasmon-induced transparency in graphene-based terahertz metamaterials, Europhys Lett, 120(2017)27005; doi.org/10.1209/0295-5075/120/27005
85. Devi K M, Chowdhury D R, Kumar G, Sarma A K, Dual-band electromagnetically induced transparency effect in a concentrically coupled asymmetric terahertz metamaterial, J Appl Phys, 124(2018)063106; doi.org/10.1063/1.5040734
86. Kadic M, Milton G W, Hecke M V, Wegener M, 3D metamaterials, Nat Rev Phys, 1(2019)198-210.
87. Cui A, Liu Z, Li J, Shen T H, Xia X, Li Z, Gong Z, Li H, Wang B, Li J, Yang H, Li W, Gu C, Directly patterned substrate-free plasmonic “nanograter” structures with unusual Fano resonances, Light Sci Appl, 4(2015)e308; doi.org/10.1038/lsa.2015.81
88. Liu Z, Du S, Cui A, Li Z, Fan Y, Chen S, Li W, Li J, Gu C, High-quality-factor mid-infrared toroidal excitation in folded 3D metamaterials, Adv Mater, 29(2017)1606298; doi.org/10.1002/adma.201606298
89. Valentine J, Zhang S, Zentgraf T, Avila E U, Genov D A, Bartal G, Zhang X, Three-dimensional optical metamaterial with a negative refractive index, Nature, 455(2008)376-379.
90. Soukoulis C M, Wegener M, Past achievements and future challenges in the development of three-dimensional photonic metamaterials, Nat Photonics, 5(2011)523-530.
91. Casse B D F, Lu W T, Huang Y J, Gultepe E, Menon L, Sridhar S, Super-resolution imaging using a three-dimensional metamaterials nanolens, Appl Phys Lett, 96(2010)023114; doi.org/10.1063/1.3291677
92. Chen H, Chan C T, Acoustic cloaking in three dimensions using acoustic metamaterials, Appl Phys Lett, 91(2007) 183518; doi.org/10.1063/1.2803315
93. Frenzel T, Kadic M, Wegener M, Three-dimensional mechanical metamaterials with a twist, Science, 358(2017) 1072-1074.
94. Liu N, Guo H, Fu L, Kaiser S, Schweizer H, Giessen H, Three-dimensional photonic metamaterials at optical frequencies, Nat Mater, 7(2008)31-37.
95. Lewin L, The electrical constants of a material loaded with spherical particles, Proc Inst Elec Eng, 94(1947)65-68.
96. Jahani S, Jacob Z, All-dielectric metamaterials, Nat Nanotechnol, 11(2016)23-36.
97. Shalaev V M, Optical negative-index metamaterials, Nat Photonics, 1(2007)41-48.
98. Ginn J C, Brener I, Peters D W, Wendt J R, Stevens J O, Hines P F, Basilio L I, Warne L K, Ihlefeld J F, Clem P G, Sinclair M B, Realizing Optical Magnetism from Dielectric Metamaterials, Phys Rev Lett, 108(2012)097402; doi.org/10.1103/PhysRevLett.108.097402
99. Gandji N P, Semouchkin G B, Semouchkina E, All-dielectric metamaterials: irrelevance of negative refraction to overlapped Mie resonances, J Phys D: Appl Phys, 50(2017)455104; doi.org/10.1088/1361-6463/aa89d3
100. Miroshnichenko A E, Kivshar Y S, Fano resonances in all-dielectric oligomers, Nano Lett, 12(2012)6459-6463.
101. Wu C, Arju N, Kelp G, Fan J A, Dominguez J, Gonzales E, Tutuc E, Brener I, Shvets G, Spectrally selective chiral silicon metasurfaces based on infrared Fano resonances, Nat Commun, 5(2014)3892; doi.org/10.1038/ncomms4892
102. Zhang J, MacDonald K F, Zheludev N I, Near-infrared trapped mode magnetic resonance in an all-dielectric metamaterial, Opt Express, 21(2013)26721-26728.
103. Zhang F, Zhao Q, Zhou J, Wang S, Polarization and incidence insensitive dielectric electromagnetically induced transparency metamaterial, Opt Express, 21(2013)19675-19680.
104. Yang Y, Kravchenko I I, Briggs D P, Valentine J, All-dielectric metasurface analogue of electromagnetically induced transparency, Nat Commun, 5(2014)5753; doi.org/10.1038/ncomms6753
105. Pu M, Song M, Yu H, Hu C, Wang M, Wu X, Luo J, Zhang Z, Luo X, Fano resonance induced by mode coupling in all-dielectric nanorods array, Appl Phys Express, 7(2014)032002; doi.org/10.7567/APEX.7.032002
106. Zhang F, Huang X, Zhao Q, Chen L, Wang Y, Li Q, He X, Li C, Chen K, Fano resonance of an asymmetric dielectric wire pair, Appl Phys Lett, 105(2014)172901; doi.org/10.1063/1.4900757
107. Fan P, Yu Z, Fan S, Brongersma M L, Optical fano resonance of an individual semiconductor nanostructure, Nat Mater, 13(2014)471-475.
108. Liu S D, Wang Z X, Wang W J, Chen J D, Chen Z H, High Q- factor with the excitation of anapole modes in dielectric split nanodisk arrays, Opt Express, 25(2017)22375-22387.
109. Filonov D S, Slobozhanyuk A P, Krasnok A E, Belov P A, Nenasheva E A, Hopkins B, Miroshnichenko A E, Kivshar Y S, Near-field mapping of fano resonances in all-dielectric oligomers, Appl Phys Lett, 104 (2014)021104; doi.org/10.1063/1.4858969
110. Chong K E, Hopkins B, Staude I, Miroshnichenko A E, Dominguez J, Decker M, Neshev D N, Brener I, Kivshar Y S, Observation of Fano resonances in all-dielectric nanoparticle oligomers, Small, 10(2014)1985-1990.
111. Kuznetsov A I, Miroshnichenko A E, Brongersma M L, Kivshar Y S, Luk’yanchuk B, Optically resonant dielectric nanostructures, Science, 354(2016) 6314 (2016)aag2472; doi.10.1126/science.aag2472
112. Campione S, Liu S, Basilio L I, Warne L K, Langston W L, Luk T S, Wendt J R, Reno J L, Keeler G A, Brener I, Sinclair M B, Broken symmetry dielectric resonators for high quality factor Fano metasurfaces, ACS Photonics, 3(2016)2362-2367.
113. Yuan S, Qiu X, Cui C, Zhu L, Wang Y, Li Y, Song J, Huang Q, Xia J, Strong Photoluminescence Enhancement in All-Dielectric Fano Metasurface with High Quality Factor, ACS Nano, 11(2017)10704-10711.
114. Yang Y, Wang W, Boulesbaa A, Kravchenko I I, Briggs D P, Puretzky A, Geohegan D, Valentine J, Nonlinear Fano-Resonant Dielectric Metasurfaces, Nano Lett, 15(2015)7388-7393.
115. Tuz V R, Khardikov V V, Kupriianov A S, Domina K L, Xu S, Wang H, Sun H B, High-quality trapped modes in all-dielectric metamaterials, Opt Express, 26(2018)2905-2916.
116. Yu P, Kupriianov A S, Dmitriev V, Tuz V R, All-dielectric metasurfaces with trapped modes: Group-theoretical description, J Appl Phys, 125(2019)143101; doi.org/10.1063/[email protected]
117. Hsu C W, Zhen B, Stone A D, Joannopoulos J D, Soljačić M, Bound states in the continuum, Nat Rev Mater, 1 (2016)16048; doi.org/10.1038/natrevmats.2016.48
118. Koshelev K, Lepeshov S, Liu M, Bogdanov A, Kivshar Y, Asymmetric metasurfaces with high-Q resonances governed by bound states in the continuum, Phys Rev Lett, 121(2018)193903; doi.org/10.1103/PhysRevLett.121.193903
119. Koshelev K, Bogdanov A, Kivshar Y, Meta-optics and bound states in the continuum, Science Bulletin, 64 (2019)836-842.
120. Koshelev K, Favraud G, Bogdanov A, Kivshar Y, Fratalocchi A, Nonradiating photonics with resonant dielectric nanostructures, Nanophotonics, 8(2019)725-745.
121. Bogdanov A A, Koshelev K L, Kapitanova P V, Rybin M V, Gladyshev S A, Sadrieva Z F, Samusev K B, Kivshar Y S, Limonov M F, Bound states in the continuum and Fano resonances in the strong mode coupling regime, Adv Photonics, 1(2019)016001; doi.org/10.1117/1.AP.1.1.016001
122. Koshelev K, Lepeshov S, Liu M, Bogdanov A, Kivshar Y, Asymmetric metasurfaces with high-Q resonances governed by bound states in the continuum, Phys Rev Lett, 121(2018)193903; doi.org/10.1103/PhysRevLett.121.193903
123. Seo B, Kim K, Kim S G, Kim A, Cho H, Choi E M, Observation of trapped-modes excited in double-layered symmetric electric ring resonators, J Appl Phys, 111(2012)113106; doi.org/10.1063/1.4721993
124. Fu T, Gao X, Xiao G, Sun T, Li Q, Zhang F, Chen Y, Li H, Deng Z L, Superlattice bilayer metasurfaces simultaneously supporting electric and magnetic Fano resonances, Opt Mater Express, 9(2019)944-952.
125. Du L H, Li J, Liu Q, Zhao J H, Zhu L G, High-Q Fano-like resonance based on a symmetric dimer structure and its terahertz sensing application, Opt Mater Express, 7(2017)1335-1342.
126. Cao T, Zhang L, Xiao Z P, Huang H, Enhancement and tunability of Fano resonance in symmetric multilayer metamaterials at optical regime, J Phys D: Appl Phys, 46(2013)39; doi. org/10.1088/0022-3727/46/39/395103
127. Wang J, Fan C, He J, Ding P, Liang E, Xue Q, Double Fano resonances due to interplay of electric and magnetic plasmon modes in planar plasmonic structure with high sensing sensitivity, Opt Express, 21(2013)2236-2244.
128. Yang S, Liu Z, Xia X, Y. E, Tang C, Wang Y, Li J, Wang L, Gu C, Excitation of ultrasharp trapped-mode resonances in mirror-symmetric metamaterials, Phys Rev B, 93, 23(2016)235407; doi.org/10.1103/PhysRevB.93.235407
129. Karmakar S, Banerjee S, Kumar D, Kamble G, Varshney R K, Chowdhury D R, Deep-Subwavelength Coupling-Induced Fano Resonances in Symmetric Terahertz Metamaterials, Physica Status Solidi (RRL), 13(2019) 201900310; doi.org/10.1002/pssr.201900310.
130. Chen H T, Padilla W J, Zide J M O, Gossard A C, Taylor A J, Averitt R D, Active Metamaterial Devices, Nature, 444 (2006)597-600.
131. Padilla W J, Taylor A J, Highstrete C, Lee M, Averitt R D, Dynamical Electric and Magnetic Metamaterial Response at Terahertz Frequencies, Phys Rev Lett, 96(2006)107401; doi.org/10.1103/PhysRevLett.96.107401
132. Chen H T, Padilla W J, Zide J M O, Bank S R, Gossard A C, Taylor A J, Averitt R D, Ultrafast optical switching of terahertz metamaterials fabricated on ErAs/GaAs nanoisland superlattices, Opt Lett, 32(2007)1620-1622.
133. Tao H, Strikwerda A C, Fan K, Padilla W J, Zhang X, Averitt R D, Reconfigurable Terahertz Metamaterials, Phys Rev Lett, 103(2009)147401; doi.org/10.1103/PhysRevLett.103.147401
134. Chowdhury D R, Singh R, O’Hara J F, Chen H T, Taylor A J, Azad A K, Dynamically reconfigurable terahertz metamaterials through photo doped semiconductors, Appl Phys Lett, 99(2011)231101; doi.org/10.1063/1.3667197
135. Zhou J, Chowdhury D R, Zhao R, Azad A K, Chen H T, Soukoulis C M, Taylor A J, O’Hara J F, Terahertz chiral metamaterials with giant and dynamically tunable optical activity, Phys Rev B, 86(2012)035448; doi.org/10.1103/PhysRevB.86.035448
136. Liu M Hwang H Y, Tao H, Strikwerda A C, Fan K, Keiser G R, Sternbach A J, West K G, Kittiwatanakul S, Lu J, Wolf S A, Omenetto F G, Zhang X, Nelson K A, Averitt R D,, Terahertz-field-induced insulator-to-metal transition in vanadium dioxide metamaterial, Nature, 487(2012)345-348.
137. Gu J, Singh R, Liu X, Zhang X, Ma Y, Zhang S, Maier S A, Tian Z, Azad A K, Chen H T, Taylor A J, Han J, Zhang W, Active control of electromagnetically induced transparency analogue in terahertz metamaterials, Nat Commun, 3(2012)1151; doi.org/10.1038/ncomms2153
138. Chowdhury D R, Singh R, Taylor A J, Chen H T, Azad A K, Ultrafast manipulation of near field coupling between bright and dark modes in terahertz metamaterial, Appl Phys Lett, 102(2013)011122; doi.org/10.1063/1.4774003
139. Cao W, Singh R, Zhang C, Han J, Tonouchi M, Zhang W, Plasmon-induced transparency in metamaterials: Active near field coupling between bright superconducting and dark metallic mode resonators, Appl Phys Lett, 103(2013) 101106; doi.org/10.1063/1.4819389
140. Heyes J E, Withayachumnankul W, Grady N K, Chowdhury D R, Azad A K, Chen H T, Hybrid metasurface for ultra-broadband terahertz modulation, Appl Phys Lett, 105(2014)181108; doi.org/10.1063/1.4901050
141. Chang W S, Lassiter J B, Swanglap P, Sobhani H, Khatua S, Nordlander P, Halas N J, Link S, A Plasmonic Fano Switch, Nano Letts, 12(2012)4977-4982.
142. Sámson Z L, MacDonald K F, Angelis F D, Gholipour B, Knight K, Huang C C, Fabrizio E D, Hewak D W, Zheludev N I, Metamaterial electro-optic switch of nanoscale thickness, Appl Phys Lett, 96 (2010)143105; doi.org/10.1063/1.3355544.
143. Xiao S, Wang T, Jiang X, Yan X, Cheng L, Wang B, Xu C, Strong interaction between graphene layer and Fano resonance in terahertz metamaterials, J Phys. D: Appl Phys, 50(2017)195101; doi.org/10.1088/1361-6463/aa69b1
144. Emani N K, Chung T F, Kildishev A V, Shalaev V M, Chen Y P, Boltasseva A, Electrical Modulation of Fano Resonance in Plasmonic Nanostructures Using Graphene, Nano Lett, 14(2014)78-82.
145. Liu B, Tang C, Chen J, Zhu M, Pei M, Zhu X, Electrically Tunable Fano Resonance from the Coupling between Interband Transition in Monolayer Graphene and Magnetic Dipole in Metamaterials, Sci Rep, 7(2017)17117; doi.org/10.1038/s41598-017-17394-y
146. Papaioannou M, Plum E, Valente J, Rogers E T F, Zheludev N I, All-optical multichannel logic based on coherent perfect absorption in a plasmonic metamaterial, APL Photonics, 1(2016)090801; doi.org/10.1063/1.4966269
147. Gu J, Singh R, Liu X, Zhang X, Ma Y, Zhang S, Maier S A, Tian Z, Azad A K, Chen H T, Taylor A J, Han J, Zhang W, Active control of electromagnetically induced transparency analogue in terahertz metamaterials, Nat Commun, 3(2012)1151; doi.org/10.1038/ncomms2153
148. Manjappa M, Srivastava Y K, Cong L, Al-Naib I A I, Singh R, Active photo switching of sharp Fano resonances in THz metadevices, Adv Mater, 29(2017)1603355; doi.org/10.1002/adma.201603355
149. Manjappa M, Pitchappa P, Singh N, Wang N, Zheludev N I, Lee C, Singh R, Reconfigurable MEMS Fano metasurfaces with multiple-input-output states for logic operations at terahertz frequencies, Nat Commun, 9(2018) 4056; doi.org/10.1038/s41467-018-06360-5
150. Fan P, Yu Z, Fan S, Brongersma M L, Optical fano resonance of an individual semiconductor nanostructure, Nat Mater, 13(2014)471-475.
151. Shcherbakov M R, Liu S, Zubyuk V V, Vaskin A, Vabishchevich P P, Keeler G, Pertsch T, Dolgova T V, Staude I, Brener I, Fedyanin A A, Ultrafast all-optical tuning of direct-gap semiconductor metasurfaces, Nat Commun, 8 (2017)17; doi.org/10.1038/s41467-017-00019-3
152. Srivastava Y K, Manjappa M, Krishnamoorthy H N S, Singh R, Accessing the high-Q dark plasmonic Fano resonances in superconductor metasurfaces, Adv Opt Mater, 4 (2016)1875-1881.
153. Srivastava Y K, Manjappa M, Cong L, Krishnamoorthy H N S, Savinov V, Pitchappa P, Singh R, A superconducting dual-channel photonic switch, Adv Mater, 30(2018)1801257; doi.org/10.1002/adma.201801257
154. Wuttig M, Bhaskaran H, Taubner T, Phase-change materials for non-volatile photonic applications, Nat Photonics, 11(2017)465-476.
155. Cao T, Wei C, Simpson R E, Zhang L, Cryan M J, Fast tuning of Fano resonance in metal/phase-change materials/metal metamaterials, Opt Mater Express, 4(2014)1775-1786.
156. Makarov S, Furasova A, Tiguntseva E, Hemmetter A, Berestennikov A, Pushkarev A, Zakhidov A, Kivshar Y, Halide-Perovskite Resonant Nanophotonics, Adv Opt Mater, 7(2019)1800784; doi.org/10.1002/adom.201800784
157. Pitchappa P, Kumar A, Prakash S, Jani H, Venkatesan T, Singh R, Chalcogenide Phase Change Material for Active Terahertz Photonics, Adv Mater, 31(2019)1808157; doi.org/10.1002/adma.201808157
158. Cong L, Xu N, Chowdhury D R, Manjappa M, Rockstuhl C, Zhang W, Singh R, Nonradiative and Radiative Resonances in Coupled Metamolecules, Adv Opt Mater, 4(2016)252-258; doi.org/10.1002/adom.201500557
Asian Journal of Physics |
Vol. 28 Nos 7-9 (2019) 689-699 |
Wavefront aberrations with incomplete Hartmann-Shack sensor data
Abbas Ommani1, Natalie Hutchings2, Ritambhar Burman3, Damber Thapa4 and Vasudevan Lakshminarayanan1
1Theoretical and Experimental Epistemology Lab, School of Optometry and Vision Science, University of Waterloo
2School of Optometry and Vision Science, University of Waterloo, Waterloo, ON., Canada
3Department of Biomedical Engineering, University of Miami, Miami, FL, USA
4Department of Mechanical Engineering, York University, Toronto, ON, Canada
Invited Article dedicated to Prof Ajoy Ghatak on his 80th Birthday
Wavefront aberrations describe the optical imperfections of the eye’s optical elements and are measured using the Hartmann Shack (HS) sensor. However, obtaining a reliable measurement of ocular aberrations can be difficult under some conditions. One specific challenge of estimating the ocular aberration clinically is when the data from the HS sensor are absent due to the opacities in the ocular components due to disease. In this paper, we studied this issue by randomly deleting a number of spots from the HS images and comparing the results with the aberration of the original HS image without the missing spots. The wavefront was derived from the HS data using Zernike polynomials. The results indicate that as much as 60 % of the HS spots can be missing without affecting the estimation of spherical defocus within typical clinically acceptable limits of ±0.25D. The results are further examined with in vivo measurements of a human eye wearing a spectacle lens simulating various models of clustered missing spots due to different disease conditions. The findings of this study provide foundational data on measuring the ocular wavefront aberrations when only a reduced HS measurement data set is available. © Anita Publications. All rights reserved.
Keywords: Wavefront aberrations, Huaneye, Hartmann-Shack sensor, Higher-order aberrations, Zernike Polynomials, Aberrometry, Clinical ophthalmology, Clinical optometry
References
1. Thapa D, Fleck A, Lakshminarayanan V, Bobier W R, Ocular wavefront aberration and refractive error in pre-school children, J Mod Opt, 58(2011)1681-1689.
2. Liang J, Grimm B, Goelz S, Bille J F, Objective measurement of wave aberrations of the human eye with the use of a Hartmann–Shack wave-front sensor, J Opt Soc Am A, 11(1994)1949-1957.
3. Lakshminarayanan V, Fleck A, Zernike polynomials: A guide, J Mod Opt, 58(2011)545-561.
4. International Organization for Standardization (ISO), Ophthalmic optics and instruments-Reporting aberrations of the human eye, Geneva, Switzerland, ISO, 2008 (ISO 24157:2008).
5. Lakshminarayanan V, Varadharajan L S, Special Functions in Optical Science and Engineering, Chapter 14, Pages 321-338, (SPIE Press, Bellingham, WA), 2015.
6. Seifert L, Tiziani H J, Osten W, Wavefront reconstruction with the adaptive Shack–Hartmann sensor, Opt Commun, 245(2005)255-269.
7. Thibos L N, Hong X, Clinical applications of the Shack-Hartmann aberrometer, Optometry and Vision Science, 76(1999)817-825.
8. Llorente L, Marcos S, Dorronsoro C, Burns S A, Effect of sampling on real ocular aberration measurements, J Opt Soc Am A, 24(2007)2783-2796.
9. Burman R, Ommani A, Thapa D, Raahemifar K, Hutchings N, Lakshminarayanan V, A Method for Estimating the Wavefront Aberrations with Missing Spot Data in a Hartmann-Shack Aberrometer. In: Advances in Optical Science and Engineering, eds. V Lakshminarayanan, I Bhattacharya, (Springer, New Delhi), 2015,pp 319-325.
10. Ommani A, Hutchings N, Thapa D, Lakshminarayanan V, Pupil scaling for the estimation of aberrations in natural pupils, Optometry and Vision Science, 91(2014)1175-1182.
11. Maeda N, Clinical applications of wavefrontaberrometry–a review, Clinical & Experimental Ophthalmology, 37(2009) 118-129.
12. Montés-Micó R, Cáliz A, Alió J L, Wavefront analysis of higher order aberrations in dry eye patients. Journal of Refractive Surgery, 20(2004)243-247.
13. Kuroda T, Fujikado T, Maeda N, Oshika T, Hirohara Y, Mihashi T, Wavefront analysis in eyes with nuclear or cortical cataract, Am J Ophthalmology, 134(2002)1-9.
Asian Journal of Physics |
Vol. 28 Nos 7-9 (2019) 701-716 |
Review of optical image encryption schemes based on fractional Hartley transform
Phool Singh1, A K Yadav2, Sunanda Vashisth3 and Kehar Singh4
1Department of Mathematics (SOET), Central University of Haryana,Mahendergarh, India 123031
2Department of Applied Mathematics, Amity University Haryana, Gurugram, India 122413
3Department of Science, DPG Degree College, Gurugram, India 122001
4Department of Applied Sciences, The North Cap University, Gurugram, India 122017
Invited Article dedicated to Prof Ajoy Ghatak on his 80th Birthday
A review of symmetric and asymmetric image encryption schemes in the fractional Hartley domain is presented in this paper. Symmetric schemes based on double random phase encoding along with chaotic transform are discussed along with flowcharts of the encryption and decryption processes. Symmetric image encryption scheme using structured phase masks based on devil’s vortex Fresnel lens is reported. Asymmetric schemes based on phase and amplitude truncation, and on modified equal modulus decomposition are also presented. Further, we discuss attacks on schemes based on the fractional Hartley transform. © Anita Publications. All rights reserved.
Keywords: Fractional Hartley transform, symmetric and asymmetric cryptosystem, image encryption, double random phase encoding.
References
1. Javidi B (Ed), Optical and Digital Techniques for Information Security, (New York: Springer-Verlag), 2005.
2. Falou A Al (Ed), Advanced Secure Optical Image Processing for Communications, (Bristol UK: IOP Publ), 2018.
3. Muniraj I, Sheridan J T,Optical Encryption and Decryption , SPIE.Spot light Vol.SL 46, e-book Bellingham SPIE Press 2019.
4. Singh K, Unnikrishnan G, Nishchal N K, Photorefractive optical processing for data security, in Photorefractive Fiber and Crystal Devices: Materials, Optical Properties, and Applications VIII. SPIE4830 (2002), p. 205-219.
5. Singh K, John R, Joseph J, Encrypted holographic memories for information security, Bull Laser Spectrosc Soc, India, 15(2005-06)1-19.
6. Alieva T, Bastiaans M J, Calvo M L, Fractional transforms in optical information processing, EURASIP J Adv Signal Process, 2005(2005)920687; doi.org/10.1155/ASP.2005.1498
7. Matoba O, Nomura T, Perez-Cabre E, Millan MS, Javidi B, Optical techniques for information security, Proc IEEE, 97(2009)1128-1148.
8. Kumar A, Singh M, Singh K, Speckle coding for optical and digital data security applications, in ‘Advances in Speckle Metrology and Related Techniques’ (Ed) Kaufmann GH Weinheim,Wiley-VCH, (2011), 239299.
9. García-varela M S M, Pérez-Cabré E, Optical data encryption, in ‘Optical and Digital Image Processing’, (eds) Crystobal G, Schelkens P, Thienpont H, (John Wiley & Sons, Ltd), 2011, pp 739-767.
10. Chen W, Javidi B, Chen X, Advances in optical security systems, Adv Opt Photon, 6(2014)120-155.
11. Liu S, Guo C, Sheridan JT, A review of optical image encryption techniques, Opt Laser Technol, 57(2014)327-342.
12. Yadav A K, Vashisth S, Singh H, Singh K, Optical cryptography and watermarking using some fractional canonical transforms and structured masks, in Adv Opt Sci Eng, Proc IEM OPTRONIX 2014, Kolkata: (eds) Lakshminarayanan V, Bhattacharya I, (Springer Proc Physics), 166(2015)25-36.
13. Kumar P, Joseph J, Singh K, Double Random Phase Encoding Based Optical Encryption Systems Using Some Linear Canonical Transforms: Weaknesses and Countermeasures, In: Healy J J, Alper Kutay M, Ozaktas H, Sheridan J (eds), Linear Canonical Transforms, (New York, NY: Springer), 2016, pp. 367-396.
14. Javidi B, Carnicer A, Yamaguchi M, Nomura T, Perez-Cabre E, Milan M, Nishchal N K, Torroba R, Barrera J F, He W, Peng X, Srern A, Rivenson Y, Alfalou A, Brosseau C, Guo C, Seridan J T, Situ G, Naruse M, Matsumoto T, Juvells I, Tajahuerce E, Lancis J, Chen W, Chen X, Pinkse P W H, Mosk A P, Markman A, Roadmap on optical security, J Opt (IOP), 18(2016)083001.
15. Singh K, Photorefractive optical cryptography: a personal tour, In: Bhattacharya I, Chakrabarti S, Reehal H S, Lakshminarayanan V, (eds), Advances in Optical Science and Engineering, Singapore, Springer (2017), 57-64.
16. Abuturab M A, Optical information security systems based on a gyrator wavelet transform, Advanced Secure Optical Image Processing for Communications, Al Falou A, (ed), Bristol UK: IOP Publ. (2018) p. 1.1-39.
17. Fatima A, Nishchal N K, Equal modulus decomposition based symmetric optical cryptosystems, Advanced Secure Optical Image Processing for Communications, Al Falou A, (ed), Bristol UK: IOP Publ. (2018) p. 5.1-15.
18. Liu Z J, Chen H, Tanougast C, Liu S, Information security using fractional transforms, Advanced Secure Optical Image Processing for Communications, Al Falou A, (ed), Bristol UK: IOP Publ. (2018) p. 6.1-32.
19. García-varela MSM, Pérez-Cabré E, Vilardy JM, Nonlinear techniques for secure optical encryption and multifactor authentication, Advanced Secure Optical Image Processing for Communications, Al Falou A, (ed), Bristol UK: IOP Publ. (2018) p. 8.1-33.
20. Tsang P W M, Enhanced single random phase holographic encryption of optical images, Advanced Secure Optical Image Processing for Communications, Al Falou A, (ed), Bristol UK: IOP Publ. (2018) p. 9.1-11.
21. Chen W, Single-pixel optical information encoding and authentication, Advanced Secure Optical Image Processing for Communications, Al Falou A, (ed), Bristol UK: IOP Publ. (2018), pp10.1-23.
22. Refregier P, Javidi B, Optical image encryption based on input plane and Fourier plane random encoding, Opt Lett, 20(1995)767-769.
23. Unnikrishnan G, Singh K, Double random fractional Fourier-domain encoding for optical security, Opt Eng, 39 (2000)2853-2859.
24. Unnikrishnan G, Joseph J, Singh K, Optical encryption by double-random phase encoding in the fractional Fourier domain, Opt Lett, 25(2000)887-889.
25. Situ G, Zhang J, Double random-phase encoding in the Fresnel domain, Opt Lett, 29(2004)1584-1586.
26. Chen L, Zhao D, Optical image encryption with Hartley transforms, Opt Lett, 31(2006)3438-3440.
27. Liu Z, Guo Q, Xu L, Ahmad MA, Liu S, Double image encryption by using iterative random binary encoding in gyrator domains, Opt Express, 18(2010)12033-12043.
28. Singh H, Yadav A K, Vashisth S, Singh K, Double phase-image encryption using gyrator transforms, and structured phase mask in the frequency plane, Opt Lasers Eng, 67(2015)145-156.
29. Joshi M, Chandrashakher, Singh K, Color image encryption and decryption using fractional Fourier transform, Opt Commun, 279(2007)35-42.
30. Kumar J, Singh P, Yadav A K, Kumar A, Asymmetric cryptosystem for phase images in fractional Fourier domain using LU-decomposition and Arnold transform, Procedia Comput Sci, 132(2018)1570-1577.
31. Singh H, Yadav AK, Vashisth S, Singh K, A cryptosystem for watermarking based on fractional Fourier transform using a random phase mask in the input plane and a structured phase mask in the frequency plane, Asian J Phys, 23(2014)597-612.
32. Singh H, Yadav A K, Vashisth S, Singh K, Double phase-image encryption using gyrator-,and fractional Fourier transforms with structured phase mask in the frequency plane followed by a gyrator transform, Asian J Phys, 24 (2015)1-16.
33. Vashisth S, Singh H, Yadav A K, Singh K, Image encryption using fractional Mellin transform, structured phase filters, and phase retrieval, Optik, 125(2014)5309-5315.
34. Vashisth S, Singh H, Yadav A K, Singh K, Devil’s vortex phase structure as frequency plane mask for image encryption using the fractional Mellin transform, Int J Opt, 2014(2014), 9 pages; doi.org/10.1155/2014/728056
35. Zhao D, Li X, Chen L, Optical image encryption with redefined fractional Hartley transform, Opt Commun, 281 (2008)5326-5329.
36. Li X, Zhao D, Optical color image encryption with redefined fractional Hartley transform, Optik, 121(2010)673-677.
37. Jimenez C, Torres C, Mattos L, Fractional Hartley transform applied to optical image encryption, J Phys Conf Ser, 274(2011)012041; doi.org/10.1088 /1742-6596/274/1/012041
38. Vilardy JM, Torres CO, Jimenez CJ, Double image encryption method using the Arnold transform in the fractional Hartley domain, Proc. SPIE 8785, 8th Iberoamerican Optics Meeting and 11th Latin American Meeting on Optics, Lasers, and Applications. SPIE Proc. (2013), p. 87851R-87851R–5.
39. Liu Y, Du J, Fan J, Gong L, Single-channel color image encryption algorithm based on fractional Hartley transform and vector operation, Multimed Tools Appl, 74(2015)3171-3182.
40. Singh H, Optical image encryption using random-vortex structured phase masks in fractional Hartley transform domain, in ‘13th International Confer on Fiber Optics and Photonics’, Kharagpur: OSA, (2016), p. Tu4A.68.
41. Singh P, Yadav A K, Singh K, Phase image encryption in the fractional Hartley domain using Arnold transform and singular value decomposition, Opt Lasers Eng, 91(2017)187-195.
42. Singh P, Yadav A K, Singh K, Saini I, Optical image encryption in the fractional Hartley domain, using Arnold transform and singular value decomposition, AIP Conf Proc, 1802(2017)020017-1–7.
43. Singh P, Yadav A, Singh K, Color image encryption using affine transform in fractional Hartley domain, Opt Appl 47(2017)421-433.
44. Yadav A K, Singh P, Singh K, Cryptosystem based on devil’s vortex Fresnel lens in the fractional Hartley domain, J Opt, 47(2018)208-219.
45. Yadav P L, Singh H, Optical double image hiding in the fractional Hartley transform using structured phase filter and Arnold transform, 3D Res, 9(2018)20; doi.org/10.1007/s13319-018-0172-0
46. Girija R, Singh H, Triple-level cryptosystem using deterministic masks and modified Gerchberg-Saxton iterative algorithm in fractional Hartley domain by positioning singular value decomposition, Optik, 187(2019)238-257.
47. Qin W, Peng X, Asymmetric cryptosystem based on phase-truncated Fourier transforms, Opt Lett, 35(2010)118-120.
48. He W, Meng X, Peng X, Asymmetric cryptosystem using random binary phase modulation based on mixture retrieval type of Yang-Gu algorithm: comment,Opt Lett, 38(2013)4044-4044.
49. Liu W, Liu Z, Liu S, Asymmetric cryptosystem using random binary phase modulation based on mixture retrieval type of Yang-Gu algorithm: reply, Opt Lett, 38(2013)4045-4045.
50. Yadav A K, Singh P, Saini I, Singh K, Asymmetric encryption algorithm for colour images based on fractional Hartley transform, J Mod Opt, 66 (2019)629-642.
51. Wang X, Zhao D, A special attack on the asymmetric cryptosystem based on phase-truncated Fourier transforms, Opt Commun, 285(2012)1078-1081.
52. Cai J, Shen X, Lei M, Lin C, Dou S, Asymmetric optical cryptosystem based on coherent superposition and equal modulus decomposition, Opt Lett, 40(2015)475-478.
53. Deng X, Asymmetric optical cryptosystem based on coherent superposition and equal modulus decomposition: comment, Opt Lett, 40(2015)3913-3913.
54. Wu J, Liu W, Liu Z, Liu S, Cryptanalysis of an asymmetric optical cryptosystem based on coherent superposition and equal modulus decomposition, Appl Opt, 54(2015)8921-8924.
55. Cai J, Shen X, Modified optical asymmetric image cryptosystem based on coherent superposition and equal modulus decomposition, Opt Laser Technol, 95(2017)105-112.
56. Singh P, Yadav A K, Singh K, Saini I, Asymmetric watermarking scheme in fractional Hartley domain using modified equal modulus decomposition, J Optoelectron Adv Mater, 21(2019)484-491.
57. Girija R, Singh H, An asymmetric cryptosystem based on the random weighted singular value decomposition and fractional Hartley domain, Multimed Tools Appl, (2019) Published online: 4 June 2019; doi:10.1007/s11042-019-7733-y.
58. Yadav P L, Singh H, Security enrichment of optical image cryptosystem based on superposition technique using fractional Hartley and gyrator transform domains deploying equal modulus decomposition, Opt Quantum Electron, 51(2019)140; doi.org/10.1007/s11082-019-1854-4
59. Singh P, Yadav A K, Singh K, Known-plaintext attack on cryptosystem based on fractional Hartley transform using particle swarm optimization algorithm, In: Ray K, Sharan S, Rawat S, Jain S, Srivastava S, Bandyopadhyay A, (eds), ‘Engineering Vibration, Communication and Information Processing’, (Singapore: Springer), 2019, pp 317-327.
60. Zhou L, Xiao X, Chen W, Machine-learning attacks on interference-based optical encryption, Opt Express, 27 (2019)26143-26154.
Asian Journal of Physics |
Vol. 28 Nos 7-9 (2019) 719-746 |
Axial vs radial junction nanowire solar cell
Vidur Raj, Hark Hoe Tan and Chennupati Jagadish
1Department of Electronic Materials Engineering, Research School of Physics,
The Australian National University, Canberra, ACT 2601, Australia
Invited Article dedicated to Prof Ajoy Ghatak on his 80th Birthday
Both axial and radial junction nanowire solar cells have their own challenges and advantages. However, so far, there is no review which explicitly provides a detailed comparative analysis of both axial and radial junction solar cells. This article reviews some of the recent results on axial and radial junction nanowire solar cells with an attempt to perform a comparative study between the optical and device behavior of these cells. In particular, we start by reviewing different results on ways in which the absorption can be tuned in axial and radial junction solar cells. We also discuss results on some of the critical device concepts that are required to achieve high efficiency in axial and radial junction solar cells. We include a section on new device concepts that can be realized in nanowire structures. Finally, we conclude this review by discussing a few of the standing challenges of nanowire solar cells. © Anita Publications. All rights reserved.
Keywords: Nanowire, Axial junction, Radial junction, III-V solar cells
References
1. Garnett E, Yang P, Light Trapping in Silicon Nanowire Solar Cells, Nano Lett, 10( 2010)1082-1087
2. Garnett E C, Brongersma M L, Cui Y, McGehee M D, Nanowire Solar Cells, Annu Rev Mater Res, 41(2011)269-295.
3. Wallentin J, Anttu N, Asoli D, Huffman M, Åberg I, Magnusson M H, Siefer G, Fuss-Kailuweit P, Dimroth F, Witzigmann B, Xu H Q, Samuelson L, Deppert K, Borgström M T, InP Nanowire Array Solar Cells Achieving 13.8% Efficiency by Exceeding the Ray Optics Limit, Science, 339(2013)1057-1060.
4. Beard M C, Luther J M, Nozik A J, The promise and challenge of nanostructured solar cells, Nat Nanotechnol, 9(2014)951-954.
5. Otnes G, Borgström M T, Towards high efficiency nanowire solar cells, Nano Today, 12(2017)31-45.
6. Tian B, Zheng X, Kempa T J, Fang Y, Yu N, Yu G, Huang J, Lieber C M, Coaxial silicon nanowires as solar cells and nanoelectronic power sources. Nature, 449(2007)885-889.
7. Kailuweit P, Peters M, Leene J, Mergenthaler K, Dimroth F, Bett A W, Numerical simulations of absorption properties of InP nanowires for solar cell applications, Prog Photovoltaics, 20(2011)945-953.
8. Gao Q, Tan H H, Jackson H E, Smith L M, Yarrison-Rice J M, Zou J, Jagadish C, Growth and properties of III–V compound semiconductor heterostructure nanowires, Semicond Sci Technol, 26(2011)014035; doi.org/10.1088/0268-1242/26/1/014035.
9. Mokkapati S, Jagadish C, III-V compound SC for optoelectronic devices, Mater Today, 12(2009)22-32.
10. Chatterjee U, Park J.-H, Um D.-Y, Lee C.-R, III-nitride nanowires for solar light harvesting: A review, Renewable and Sustainable Energy Reviews, 79(2017)1002-1015.
11. Joyce H J, Gao Q, Hoe Tan H, Jagadish C, Kim Y, Zou J, Smith L M, Jackson H E, Yarrison-Rice J M,Parkinson P, Johnston M B, III–V semiconductor nanowires for optoelectronic device applications, Prog Quantum Electron, 35(2011)23-75.
12. Li Z,Tan H H, Jagadish C, Fu L, III–V Semiconductor Single Nanowire Solar Cells: A Review, Adv Mater Tech, 3(2018)1800005; doi.org/10.1002 /admt.201800005
13. Tedeschi D, De Luca M, Fonseka H A, Gao Q, Mura F, Tan H H, Rubini S, Martelli F, Jagadish C, Capizzi M, Polimeni A, Long-Lived Hot Carriers in III-V Nanowires, Nano Lett, 16(2016)3085-3093.
14. Mokkapati S, Jagadish C, Review on photonic properties of nanowires for photovoltaics Invited, Opt Express, 24(2016) 17345-17358.
15. Raj V, Fu L,Tan H H, Jagadish C, Design Principles for Fabrication of InP-Based Radial Junction Nanowire Solar Cells Using an Electron Selective Contact, IEEE J Photovolt, 9(2019)980-991.
16. Li Z, Wenas Y C, Fu L, Mokkapati S, Tan H H, Jagadish C, Influence of Electrical Design on Core–Shell GaAs Nanowire Array Solar Cells, IEEE J Photovolt, 5(2015)854-864.
17. Yoon J, Sun Y, Rogers J A, Chapter 6 – Flexible Solar Cells Made of Nanowires/Microwires. In Semiconductor Nanomaterials for Flexible Technologies, (eds) Sun Y, Rogers J A, (William Andrew Publishing, Oxford), 2010, pp 159-196.
18. Levine A, Yuan G B, Xie J, Wang D W, Preparations and Energetic Applications of Si Nanowires, Sci Adv Mater, 2(2010)463-473.
19. Fan Z, Ruebusch D J, Rathore A A, Kapadia R, Ergen O, Leu P W, Javey A, Challenges and prospects of nanopillar-based solar cells, Nano Res, 2(2009)829-843.
20. Hsu C H, Wu J R, Lu Y T, Flood D J, Barron A R, Chen L C, Fabrication and characteristics of black silicon for solar cell applications: An overview, Mater Sci Semicond, 25(2014)2-17.
21. Kapadia R, Fan Z Y, Takei K, Javey A, Nanopillar photovoltaics: Materials, processes, and devices, Nano Energy, 1(2012)132-144.
22. LaPierre R R, Chia A C E, Gibson S J, Haapamaki C M, Boulanger J, Yee R, Kuyanov P, Zhang J, Tajik N, Jewell N, Rahman K M A, III–V nanowire photovoltaics: Review of design for high efficiency, Phys Status Solidi-R, 7 (2013)815-830.
23. Li J S, Yu H Y, Li Y L, Aligned Si nanowire-based solar cells, Nanoscale, 3(2011)4888-4900.
24. Mann J K, Kurstjens R, Pourtois G, Gilbert M, Dross F, Poortmans J, Opportunities in nanometer sized Si wires for PV applications, Prog Mater Sci, 58(2013)1361-1387.
25. Narasimhan V K, Cui Y, Nanostructures for photon management in solar cells, Nanophotonics, 2(2013)187-210.
26. Yiyan C, Zhiming W, Jianchao N, Waseem A B, Jing L, Shuping L, Kai H, Junyong K, Type-II Core/Shell Nanowire Heterostructures and Their Photovol-taic Applications, Nano-Micro Lett, 4(2012)135-141.
27. Yu K, Chen J, Enhancing Solar Cell Efficiencies through 1-D Nanostructures, Nanoscale Res Lett, 4(2008)1; doi.org/10.1007%2Fs11671-008-9200-y.
28. Sharma R, Dusad L K, A review and analysis on growth and optical absorption properties of silicon nanowire array for photovoltaic applications, Mod Phys Lett B, 29(2015)1530007; doi.org/10.1142/S0217984915300070.
29. Zhang Y Y, Liu H Y, Nanowires for High-Efficiency, Low-Cost Solar Photovoltaics, Crystals, 9(2019)25; doi.org/10.3390/cryst9020087
30. Zhu J, Yu Z F, Fan S H, Cui Y, Nanostructured photon management for high performance solar cells, Mat Sci Eng R, 70(2010)330-340.
31. Zhou K Y, Guo Z Y, Liu S T, Lee J H, Current Approach in Surface Plasmons for Thin Film and Wire Array Solar Cell Applications, Materials, 8(2015)4565-4581.
32. Sahoo M K, Kale P, Integration of silicon nanowires in solar cell structure for efficiency enhancement: A review. J Materiomics, 5(2019)34-48.
33. Haverkort J E M, Garnett E C, Bakkers E P A M, Fundamentals of the nanowire solar cell: Optimization of the open circuit voltage, Appl Phys Rev, 5(2018)031106; doi.org/10.1063/1.5028049.
34. Sun J K, Jiang Y, Zhong X H, Hu J S, Wan L J, Three-dimensional nanostructured electrodes for efficient quantum- dot-sensitized solar cells, Nano Energy, 32(2017)130-156.
35. Sun K, Kargar A, Park N, Madsen K N, Naughton P W, Bright T, Jing Y, Wang D L, Compound Semiconductor Nanowire Solar Cells, IEEE J Sel Top Quantum Electron, 17(2011)1033-1049.
36. Hyun J K, Zhang S, Lauhon L J, Nanowire Heterostructures, Annu Rev Mater Res, 43(2013)451-479.
37. Kupec J, Stoop R L, Witzigmann B, Light absorption and emission in nanowire array solar cells, Opt Express, 18(2010)27589-27605.
38. Zhang Y, Wu J, Aagesen M, Liu H, III–V nanowires and nanowire optoelectronic devices. J Phys D: Appl Phys, 48(2015)463001; doi.org/10.1088/0022-3727/48/46/463001.
39. Heiss M, Russo-Averchi E, Dalmau-Mallorquí A,Tütüncüoğlu G, Matteini F, Rüffer D, Conesa-Boj S, Demichel O, Alarcon-Lladó E, Fontcuberta i Morral A, III–V nanowire arrays: growth and light interaction, Nanotechnology, 25(2013)014015; doi.org/10.1088/0957-4484/25/1/014015
40. Chen W, Roca I Cabarrocas P, Rational design of nanowire solar cells: from single nanowire to nanowire arrays,Nanotechnology, 30(2019)194002; doi.org/10.1088/1361-6528/aaff8d
41. Goktas N I, Wilson P, Ghukasyan A, Wagner D, McNamee S, LaPierre R R, Nanowires for energy: A review, Appl Phys Rev, 5(2018)041305; doi.org/10.1063/1.5054842
42. Krogstrup P, Jørgensen H I, Heiss M, Demichel O, Holm J V, Aagesen M, Nygard J, Fontcuberta i Morral, A, Single-nanowire solar cells beyond the Shockley–Queisser limit, Nat Photonics, 7(2013)306-310.
43. Mokkapati S, Catchpole K R, Nanophotonic light trapping in solar cells, J Appl Phys, 112(2012)101101; doi.org/10.1063/1.4747795.
44. Mokkapati S, Jagadish C, Review on photonic properties of nanowires for photovoltaics Invited, Opt Express, 24(2016)17345; doi.org/10.1364/OE.24.017345
45. Otnes G, Borgström M T, Towards high efficiency nanowire solar cells, Nano Today, 12(2017)31-45.
46. Kempa T J, Day, R W, Kim S.-K, Park H.-G, Lieber C M, Semiconductor nanowires: a platform for exploring limits and concepts for nano-enabled solar cells, Energy Environ Sci, 6(2013)719-733.
47. Brongersma M L, Cui Y, Fan S, Light management for photovoltaics using high-index nanostructures, Nat Mater, 13(2014)451-460.
48. Abujetas D R, Paniagua-Domínguez R, Sánchez-Gil J A, Unraveling the Janus Role of Mie Resonances and Leaky/Guided Modes in Semiconductor Nanowire Absorption for Enhanced Light Harvesting, ACS Photonics, 2(2015)921-929.
49. Cao L, White J S, Park J.-S, Schuller J A, Clemens B M, Brongersma M L, Engineering light absorption in semiconductor nanowire devices, Nat Mater, 8(2009)643-647.
50. Hong S, Bae J, Koo B, Chang I, Cho G Y, Kim Y B, Cha S W, Prinz F B, Nanostructuring Methods for Enhancing Light Absorption Rate of Si-Based Photovoltaic Devices: A Review, Int J Precis Eng Manuf-Green Technol, 1(2014)67-74.
51. Mann S A, Grote R R, Osgood R M, Alù A, Garnett E C, Opportunities and Limitations for Nanophotonic Structures to Exceed the Shockley–Queisser Limit, ACS Nano, 10(2016)8620-8631.
52. Zeng Y, Ye Q, Shen W, Design principles for single standing nanowire solar cells: going beyond the planar efficiency limits, Sci Rep, 4(2014)4915; doi.org/10.1038/srep04915.
53. Anttu N, Xu H Q, Efficient light management in vertical nanowire arrays for photovoltaics. Opt Express, 21(2013) A558-A575.
54. Chen Y, Kivisaari P, Pistol M E, Anttu N, Optimization of the short-circuit current in an InP nanowire array solar cell through opto-electronic modeling, Nanotechnology, 27(2016) 9; doi.org/10.1088/0957-4484/27/43/435404 .
55. Cao L, Fan P, Vasudev A P, White J S, Yu Z, Cai W, Schuller J A, Fan S, Brongersma M L, Semiconductor Nanowire Optical Antenna Solar Absorbers. Nano Lett,10(2010)439-445.
56. Sturmberg B C P, Dossou K B, Botten L C, Asatryan A A, Poulton C G, McPhedran R C, de Sterke C M, Optimizing Photovoltaic Charge Generation of Nanowire Arrays: A Simple Semi-Analytic Approach, ACS Photonics, 1(2014)683-689.
57. Yu Y, Cao L, Leaky mode engineering: A general design principle for dielectric optical antenna solar absorbers, Opt Commun, 314(2014)79-85.
58. Wu D, Tang X, Wang K, Li X, An Analytic Approach for Optimal Geometrical Design of GaAs Nanowires for Maximal Light Harvesting in Photovoltaic Cells, Sci Rep, 7(2017)46504; https://doi.org/10.1038/srep46504
59. Wu D, Tang X, Wang K, He Z, Li X, An Efficient and Effective Design of InP Nanowires for Maximal Solar Energy Harvesting, Nanoscale Res Lett, 12(2017)604-604.
60. Gibson S J, van Kasteren B, Tekcan B, Cui Y, van Dam D, Haverkort J E M, Bakkers E P A M, Reimer M E, Tapered InP nanowire arrays for efficient broadband high-speed single-photon detection, Nat Nanotechnol, 14(2019) 473-479.
61. Zhang J, Ai L, Yan X, Wu Y, Wei W, Zhang M, Zhang X, Photovoltaic Performance of Pin Junction Nanocone Array Solar Cells with Enhanced Effective Optical Absorption. Nanoscale Res Lett, 13(2018)306; doi.org/10.1186/s11671-018-2727-7
62. Wang B, Stevens E, Leu P W, Strong broadband absorption in GaAs nanocone and nanowire arrays for solar cells. Opt Express, 22(2014)A386-A395.
63. Joshi M, Raj V, Balaji S P, Kaushik A, Ag–ZnO Nanocomposite for Multi Gas Sensing Applications. In Physics of Semiconductor Devices, (eds) Jain V K,Verma A, (Springer International Publishing; Cham), 2014, pp 453-456.
64. Raj V, Balaji S P, Joshi M, Kumar M, Ag Grafted ZnO Nanoplates for Photocatalytic Applications, Mater Focus, 3(2014)385-391.
65. Pescaglini A, Iacopino D, Metal nanoparticle–semiconductor nanowire hybrid nanostructures for plasmon-enhanced optoelectronics and sensing, J Mater Chem, C 3(2015)11785-11800.
66. Yen Y.-C, Chen J.-A, Ou S, Chen Y.-S, Lin K.-J, Plasmon-Enhanced Photocurrent using Gold Nanoparticles on a Three-Dimensional TiO2 Nanowire-Web Electrode, Sci Rep, 7(2017)42524, doi.org/10.1038/srep42524.
67. Li Y, Yan X, Wu Y, Zhang X, Ren X, Plasmon-Enhanced Light Absorption in GaAs Nanowire Array Solar Cells, Nanoscale Res Lett, 10(2015)436; doi.org/10.1186/s11671-015-1110-1.
68. Zhong Z, Li Z, Gao Q, Li Z, Peng K, Li L, Mokkapati S,Vora K,Wu J, Zhang G,Wang Z, Fu L,Tan H H, Jagadish C, Efficiency enhancement of axial junction InP single nanowire solar cells by dielectric coating, Nano Energy, 28(2016)106-114.
69. Anttu N, Namazi K L, Wu P M,Yang P, Xu H, Xu H Q, Håkanson U, Drastically increased absorption in vertical semiconductor nanowire arrays: A non- absorbing dielectric shell makes the difference, Nano Res, 5(2012)863-874.
70. Kim S.-K, Zhang X, Hill D J, Song K.-D, Park J.-S, Park H.-G, Cahoon J F. Doubling Absorption in Nanowire Solar Cells with Dielectric Shell Optical Antennas, Nano Lett, 15(2015)753-758.
71. Grandidier J, Callahan D M, Munday J N, Atwater H A, Gallium Arsenide Solar Cell Absorption Enhancement Using Whispering Gallery Modes of Dielectric Nanospheres, IEEE J Photovolt, 2(2012)123-128.
72. Wu D, Tang X, Wang K, Li X, Effective coupled optoelectrical design method for fully infiltrated semiconductor nanowires based hybrid solar cells, Opt Express, 24(2016)A1336-A1348.
73. Wu Y, Yan X, Wei W, Zhang J, Zhang X, Ren X, Optimization of GaAs Nanowire Pin Junction Array Solar Cells by Using AlGaAs/GaAs Heterojunctions, 13(2018), doi.org/10.1186/s11671-018-2503-8.
74. Sanatinia R, Awan K M, Naureen S, Anttu N, Ebraert E, Anand S, GaAs nanopillar arrays with suppressed broadband reflectance and high optical quality for photovoltaic applications, Opt Mater Express, 2(2012)1671-1679.
75. Huang N, Povinelli M L, Design of Passivation Layers on Axial Junction GaAs Nanowire Solar Cells, IEEE J Photovolt, 4(2014)1511-1517.
76. Raj V, Vora K, Li L, Fu L, Tan H H, Jagadish C, Electron selective contact for high efficiency core-shell nanowire solar cell, ACS Nano Article ASAP; doi.org/10.1021/acsnano.9b06226
77. Shockley W, Queisser H J, Detailed Balance Limit of Efficiency of p-n Junction Solar Cells, J Appl Phys, 32(1961) 510-519.
78. Anttu N, Shockley–Queisser Detailed Balance Efficiency Limit for Nanowire Solar Cells, ACS Photonics, 2(2015) 446-453.
79. Xu Y, Gong T, Munday J N, The generalized Shockley-Queisser limit for nanostructured solar cells, Sci Rep, 5(2015)13536; doi.org/10.1038/srep13536
80. van Dam D, van Hoof N J J, Cui Y, van Veldhoven P J, Bakkers E P A M, Gómez Rivas J, Haverkort J E M, High-Efficiency Nanowire Solar Cells with Omnidirectionally Enhanced Absorption Due to Self-Aligned Indium–Tin–Oxide Mie Scatterers, ACS Nano, 10(2016)11414-11419.
81. Braun A, Katz E A, Feuermann D, Kayes B M, Gordon J M, Photovoltaic performance enhancement by external recycling of photon emission, Energy & Environmental Science, 6(2013)1499-1503.
82. Kosten E D, Kayes B M, Atwater H A, Experimental demonstration of enhanced photon recycling in angle-restricted GaAs solar cells, Energy Environ Sci, 7(2014)1907-1912.
83. Campbell P, Green M A, The limiting efficiency of silicon solar cells under concentrated sunlight, IEEE Trans Electron Devices, 33(1986)234-239.
84. Gao Q, Li Z, Li L,Vora K, Li Z, Alabadla A, Wang F, Guo Y, Peng K, Wenas Y C, Mokkapati S, Karouta F, Tan H H, Jagadish C, Fu L, Axial p-n junction design and characterization for InP nanowire array solar cells, Prog Photovoltaics, 27(2019)237-244.
85. Otnes G, Barrigón E, Sundvall C, Svensson K E, Heurlin M, Siefer G, Samuelson L, Åberg I, Borgström M T, Understanding InP Nanowire Array Solar CellPerformance by Nanoprobe-Enabled Single Nanowire Measurements, Nano Lett, 18(2018)3038-3046.
86. Chen Y, Kivisaari P, Pistol M E, Anttu N, Optimized efficiency in InP nanowire solar cells with accurate 1D analysis, Nanotechnology, 29(2018)8,doi.org/10.1088/1361-6528/aa9e73.
87. Raj V, Santos T S D, Rougieux F, Vora K, Lysevych M, Fu L, Mokkapati S,Tan H H, Jagadish C, Indium phosphide based solar cell using ultra-thin ZnO as an electron selective layer, J Phys D: Appl Phys, 51(2018)395301; doi.org/10.1088/1361-6463/aad7e3
88. Åberg I, Vescovi G, Asoli D, Naseem U, Gilboy J P, Sundvall C, Dahlgren A, Svensson K E, Anttu N, Björk M T, Samuelson L, A GaAs Nanowire Array Solar Cell With 15.3% Efficiency at 1 Sun, IEEE J Photovolt, 6(2016)185-190.
89. Anttu N, Physics and design for 20% and 25% efficiency nanowire array solar cells, Nanotechnology, 30(2018) 074002; doi.org/10.1088/1361-6528/aaf3f5
90. Wang X, Khan M R, Lundstrom M, Bermel P, Performance-limiting factors for GaAs-based single nanowire photovoltaics, Opt Express, 22(2014)A344-A358.
91. Lee K, Hwang I, Kim N, Choi D, Um H.-D, Kim S, Seo K, 17.6%-Efficient radial junction solar cells using silicon nano/micro hybrid structures, Nanoscale, 8(2016)14473-14479.
92. Oener S Z, Cavalli A, Sun H, Haverkort J E M, Bakkers E P A M, Garnett E C, Charge carrier-selective contacts for nanowire solar cells, Nature Commun, 9(2018)3248; doi.org/10.1038/s41467-018-05453-5
93. Maryasin V, Bucci D, Rafhay Q, Panicco F, Michallon J, Kaminski-Cachopo A, Technological guidelines for the design of tandem III-V nanowire on Si solar cells from opto-electrical simulations, Sol Energy Mater Sol Cells, 172(2017)314-323.
94. Tajik N, Haapamaki C M, LaPierre R R, Photoluminescence model of sulfur passivated p-InP nanowires, Nanotechnology, 23(2012)315703; doi.org/10.1088/0957-4484/23/31/315703.
95. Tajik N, Peng Z, Kuyanov P, LaPierre R R, Sulfur passivation and contact methods for GaAs nanowire solar cells, Nanotechnology, 22(2011)225402; doi.org/10.1088/0957-4484/22/22/225402
96. Naureen S, Shahid N, Sanatinia R, Anand S, Top-Down Fabrication of High Quality III–V Nanostructures by Monolayer Controlled Sculpting and Simultaneous Passivation. Adv Funct Mater, 23(2013)1620-1627.
97. Black L E, Cavalli A, Verheijen M A, Haverkort J E M, Bakkers E P A M, Kessels W M M, Effective Surface Passivation of InP Nanowires by Atomic-Layer- Deposited Al2O3 with POx Interlayer, Nano Lett, 17(2017)6287-6294.
98. Ren D, Azizur-Rahman K M, Rong Z, Juang B.-C, Somasundaram S, Shahili M, Farrell A C, Williams B S, Huffaker D L, Room-Temperature Midwavelength Infrared InAsSb Nanowire Photodetector Arrays with Al2O3 Passivation, Nano Lett, 19(2019)2793-2802.
99. Holm J V, Jørgensen H I, Krogstrup P, Nygård J, Liu H, Aagesen M, Surface-passivated GaAsP single-nanowire solar cells exceeding 10% efficiency grown on silicon, Nature Commun, 4(2013)1498; doi.org/10.1038/ncomms2510
100. Cui Y, Plissard S, Wang J, Vu T T T, Smalbrugge E, Geluk E J, de Vries T, Bolk J, Trainor M, Verheijen M A, Haverkort J E M, Bakkers E, Ieee InP nanowire array solar cell with cleaned sidewalls, In 2013 IEEE 39th Photovoltaic Specialists Conference, New York, 2013; pp 3389-3392.
101. Cui Y C,Wang J, Plissard S R, Cavalli A, Vu T T T, van Veldhoven R P J, Gao L, Trainor M, Verheijen M A, Haverkort J E M, Bakkers E, Efficiency Enhancement of InP Nanowire Solar Cells by Surface Cleaning, Nano Lett, 13(2013)4113-4117.
102. Li Y, Chen Q, He D, Li J, Radial junction Si micro/nano-wire array photovoltaics: Recent progress from theoretical investigation to experimental realization. Nano Energy, 7(2014)10-24.
103. Kayes B M, Atwater H A, Lewis N S, Comparison of the device physics principles of planar and radial p-n junction nanorod solar cells, J Appl Phys, 97(2005)114302; doi.org/10.1063/1.1901835
104. Borblik V, Electrostatics of Nanowire Radial p–n Heterojunctions, J Electron Mater, 47(2018)4022-4027.
105. Chia A C E, La Pierre R R, Electrostatic model of radial pn junction nanowires, J Appl Phys,114(2013)074317; doi.org/10.1063/1.4818958
106. Mariani G, Zhou Z, Scofield A, Huffaker D L, Direct-Bandgap Epitaxial Core–Multishell Nanopillar Photovoltaics Featuring Subwavelength Optical Concentrators, Nano Lett, 13(2013)1632-1637.
107. Heurlin M, Magnusson M H, Lindgren D, M Ek, Wallenberg L R, Deppert K, Samuelson L, Continuous gas-phase synthesis of nanowires with tunable properties, Nature, 492(2012)90-94; doi.org/10.1038/nature11652
108. Barrigón E, Hultin O, Lindgren D, Yadegari F, Magnusson M H, Samuelson L, Johansson L I M, Björk M T, GaAs Nanowire pn-Junctions Produced by Low- Cost and High-Throughput Aerotaxy, Nano Lett, 18(2018)1088-1092.
109. Barrigón E, Heurlin M, Bi Z, Monemar B, Samuelson L, Synthesis and Applications of III–V Nanowires, Chem Rev, 119(2019)9170-9220; doi.10.1021/acs.chemrev.9b00075.
110. Metaferia W, Sivakumar S, Persson A R, Geijselaers I,Wallenberg L R, Deppert K, Samuelson L, Magnusson M H, n-type doping and morphology of GaAs nanowires in Aerotaxy, Nanotechnology, 29(2018)285601; doi: 10.1088/1361-6528/aabec0
111. Zhang W,Yang F, Messing M E, Mergenthaler K, Pistol M.-E, Deppert K, Samuelson L, Magnusson M H, Yartsev A, Recombination dynamics in aerotaxy- grown Zn-doped GaAs nanowires. Nanotechnology, 27(2016)455704; doi.org/10.1088/0957-4484/27/45/455704.
112. Chen Y, Pistol M E, Anttu N, Design for strong absorption in a nanowire array tandem solar cell, Sci Rep, 6(2016) 32349; doi: 10.1038/srep32349 (2016).
113. Yao M, Cong S, Arab S, Huang N, Povinelli M L, Cronin S B, Dapkus P D, Zhou C, Tandem Solar Cells Using GaAs Nanowires on Si: Design, Fabrication, and Observation of Voltage Addition, Nano Lett, 15(2015)7217-7224.
114. Borgström M T, Magnusson M H, Dimroth F, Siefer G, Höhn O, Riel H, Schmid H, Wirths S, Björk M, Åberg I, Peijnenburg W, Vijver M, Tchernycheva M, Piazza V, Samuelson L, Towards Nanowire Tandem Junction Solar Cells on Silicon, IEEE J Photovolt, 8(2018)733-740.
115. Heurlin M, Wickert P, Fält S, Borgström M T, Deppert K, Samuelson L, Magnusson M H, Axial InP Nanowire Tandem Junction Grown on a Silicon Substrate, Nano Lett,11(2011)2028-2031.
116. Maryasin V, Rafhay, Q.;Bucci, D.;Michallon, J.; Kaminski-Cachopo, A. Opto-electrical simulation of III-V nanowire based tandem solar cells on Si. AIP Conf Procs, 1999(2018)120001; doi. org/10.1063/1.5049318
117. Wang S, Yan X, Zhang X, Li J, Ren X, Axially connected nanowire core-shell p-n junctions: a composite structure for high-efficiency solar cells, Nanoscale Research Letters, 10(2015)22; doi. org/10.1186/s11671-015-0744-3
118. Mohseni P K, Behnam A, Wood J D, English C D, Lyding J W, Pop E, Li X, InxGa1–xAs Nanowire Growth on Graphene: van der Waals Epitaxy Induced Phase Segregation, Nano Lett, 13(2013)1153-1161.
119. Hong Y J, Lee W H,Wu Y, Ruoff R S, Fukui
Axial vs radial junction nanowire solar cell.pdf
Vidur Raj, Hark Hoe Tan and Chennupati Jagadish
Asian Journal of Physics |
Vol. 28 Nos 7-9 (2019) 749-759 |
Design, characterisation and optimisation of silicon-on-insulator (SOI) based photonic devices
Weifeng Jiang1, Souvik Ghosh2, and B M A Rahman2
1College of Electronic and Optical Engineering,
Nanjing University of Posts and Telecommunications, Nanjing, China.
2City University of London, United Kingdom, EC1V 0HB
Invited Article dedicated to Prof Ajoy Ghatak on his 80th Birthday
In this paper, we review several recent works on the silicon-on-insulator based nanometre scaled photonic devices. First, we present three novel designs of SOI-based power splitter, polarisation splitter, and spot-size converter and their working efficiencies. Next, a smart design of horizontal slot hybrid plasmonic waveguide incorporated asymmetric Mach-Zehnder interferometer (A-MZI) is reported as an efficient temperature sensor where the deterioration of the interference fringe visibility has been compensated by using an unequal power splitting configuration. The synergy of high index contrast of the SOI platform and comprehensive design analyses make them attractive for the on-chip photonic device applications. © Anita Publications. All rights reserved.
Keywords: Silicon photonics, Finite element method (FEM), modal solutions, LSBR, photonic devices
References
1. Soref R, Larenzo J, All-silicon active and passive guided-wave components for λ = 1.3 and 1.6 µm, IEEE J Quantum Electron, 22(1986)873-879.
2. Jin J M, The finite element method in electromagnetics, ( John Wiley & Sons), 2015.
3. Lee J F, Finite element analysis of lossy dielectric waveguides, IEEE Transaction Microwave Theory and Techniques, 42(1994)1025-1031.
4. Rahman B M A, Davies J B, Finite-element solution of integrated optical waveguides, J Lightwave Technol, 2(1984) 682-688.
5. Rahman B M A, Davies J B, Analysis of optical waveguide discontinuities, J Lightwave Technol, 6(1988)52-57.
6. Lagali N S, Paiam M R, Macdonald R I, Rhoff K, Driessen A, Analysis of generalized Mach-Zehnder interferometers for variable-ratio power splitting and optimized switching, J Lightwave Technol, 17(1999)2542-2550.
7. Feng D J, Lay T S, Compact multimode interference couplers with arbitrary power splitting ratio, Opt Express, 16(2008)7175-7180.
8. Le T T, Cahill L W, Elton D M, Design of 2 × 2 SOI MMI couplers with arbitrary power coupling ratios, Electron Lett, 45(2009)1118-1119.
9. Cherchi M, Ylinen S, Harjanne M, Kapulainen M, Vehmas T, Aalto T, Unconstrained splitting ratios in compact double-MMI couplers, Opt Express, 22(2014)9245-9253.
10. Jiang W, Rahman B M A, Design of power-splitter with selectable splitting-ratio using angled and cascaded MMI-coupler, IEEE J Quantum Electron, 54(2018)6300509; doi: 10.1109/JQE.2018.2874087
11. Jiang W, Sun X, Rahman B M A, Compact and fabrication-tolerant polarization splitter based on horizontal triple-slot waveguide, Appl Opt, 56(2017)2119-2126.
12. Cheng N, Ma Y, Fu P, Chin C, Huang D, Horizontal slot waveguides for polarization branching control, Appl Opt, 54(2015)436-443.
13. Xiao J, Liu X, Sun X, Design of a compact polarization splitter in horizontal multiple-slotted waveguide structures, Jpn J Appl Phys, 47(2008)3748-3754.
14. Bozzola A, Carroll L, Gerace D, Cristiani I, Andreani L C, Optimising apodized grating couplers in a pure SOI platform to –0.5 dB coupling efficiency, Opt Express, 23(2015)16289-16304.
15. Pu M, Liu L, Ou H, Yvind K, Hvam J M, Ultra-low-loss inverted taper coupler for silicon-on-insulator ridge waveguide, Opt Commun, 283(2010)3678-682.
16. Loh T H, Wang Q, Zhu J, Ng K T, Lai Y C, Huang Y, Ho S T, Ultra-compact multilayer Si/SiO2 GRIN lens mode-size converter for coupling single-mode fiber to Si-wire waveguide, Opt Express, 18(2010)21519-21533.
17. Yoda Hand Shiraishi K, A new scheme of a lensed fiber employing a wedge-shaped graded-index fiber tip for the coupling between high-power laser diodes and single-mode fibers, J Lightwave Technol, 19(2001)1910-1917.
18. Barkai A, Liu A, Kim D, Cohen R, Elek N, Chang H H, Malik B H, Gabay R, Jones R, Paniccia M, Izhaky N, Double-stage taper for coupling between SOI waveguides and single-mode fiber, J Lightwave Technol, 26(2008)3860-3865.
19. Yoshida T, Omoda E, Atsumi Y, Nishi T, Tajima S, Miura N, Mori M, Sakakibara Y, Vertically curved Si waveguide coupler with low loss and flat wavelength window, J Lightwave Technol, 34(2016)1567-1571.
20. Jiang W, Kohli N, Sun X, Rahman B M A, Multi-poly-silicon-layer based spot-size converter for efficient coupling between silicon waveguide and standard single-mode fiber, IEEE Photon J, 8(2016)6600612; doi:10.1109/JPHOT.2016.2577594.
21. Jiang W, Rahman B M A, Phase-matched multi-layer based polarisation-independent spot-size converter for silicon nanowire, Sci Rep, 9(2019)12362; doi.org/10.1038/s41598-019-48848-0
22. Ghosh S, Rahman B M A, Evolution of plasmonic modes in a metal nano-wire studied by a modified finite element method, J LightwaveTechnol, 36(2017)809-818; doi.10.1109/JLT.2017.2782710
23. Ghosh S, Rahman B M A, Design of on-chip hybrid plasmonic Mach-Zehnder interferometer for temperature and concentration detection of chemical solution, Sensors and Actuators B: Chemical, 279(2019)490-502.
Asian Journal of Physics |
Vol. 28 Nos 7-9 (2019) 761-774 |
Are optical quantum information processing experiments possible without beamsplitter?
Kishore Thapliyal1 and Anirban Pathak2
1RCPTM, Joint Laboratory of Optics of Palacky University and
Institute of Physics of Academy of Science of the Czech Republic, Faculty of Science, Palacky University,
17. listopadu 12, 771 46 Olomouc, Czech Republic
2Jaypee Institute of Information Technology, A-10, Sector-62, Noida, UP-201309, India
Invited Article dedicated to Prof Ajoy Ghatak on his 80th Birthday
The significance of beamsplitter in experimental optical quantum information processing and quantum technology is discussed with a focus on the role of a beamsplitter-type Hamiltonian in the recent development in this field of research. Here, we follow a new approach to briefly describe quantum measurement, Bell measurement, quantum state engineering, quantum teleportation, cryptography, and computation using both discrete and continuous variables to establish the wide applications of beamsplitter-type operation. Finally, we also discuss the limitations of this linear optical element. © Anita Publications. All rights reserved.
Keywords: Beamsplitter operation, quantum computation, quantum communication, quantum state engineering, ap- plications of beamsplitter operation
References
1. Ghatak A, Thyagarajan K, An introduction to fiber optics, (Cambridge University Press, New York), 1998.
2. Ghatak A, Lokanathan S, Quantum mechanics: theory and applications, (Kluwer Academic Publishers, Dordrecht), 2004.
3. Ghatak A, Optics, (McGraw-Hill, New Delhi), 2005.
4. Ghatak A, Experiments with Light, (M V Learning, New Delhi), 2015.
5. Diggavi S, Thyagarajan K, Ghatak A, Perturbation analysis of polarization splitting directional couplers, App Opt, 28(1989)3450-3455.
6. Thyagarajan K, Ghatak A, Quantum theory of interaction of radiation field with matter, Lasers,(Springer), 2011, pp 225-261.
7. Aasi J, Abadie J, Abbott B P, Abbott R, Abbott T D, Abernathy M R, Adams C, Adams T, Addesso P, Adhikari R X, et al, Enhanced sensitivity of the LIGO gravitational wave detector by using squeezed states of light, Nature Photonics, 7(2013)613-619; doi.org/10.1038/nphoton.2013.177
8. Grote H, Danzmann K, Dooley K L, Schnabel R, Slutsky J, Vahlbruch H, First long-term application of squeezed states of light in a gravitational-wave observatory, Phys Rev Lett, 110(2013)181101; doi.org/10.1103/PhysRevLett.110.181101
9. Pathak A, Ghatak A (eds), Lecture on Quantum Mechanics: Fundamentals and Applications, (Viva Books, New Delhi), 2019.
10. Salih H, Li ZH, Al-Amri M, Zubairy M S, Protocol for direct counterfactual quantum communication, Phys Rev Lett, 110(2013)170502; doi.org/10.1103PhysRevLett.110.170502
11. Herrero-Collantes M, Garcia-Escartin J C, Quantum random number generators, Rev Mod Phys, 89(2017)015004; doi.org/10.1103/RevModPhys.89.015004
12. Brown R H, Twiss R Q, Correlation between photons in two coherent beams of light, Nature, 177(1956)27-29.
13. Fox M, Quantum Optics: An Introduction, (Oxford University Press, Oxford), 2006.
14. Breitenbach G, Schiller S, Mlynek J, Measurement of the quantum states of squeezed light, Nature, 387(1997)471-475.
15. Bachor H A, Ralph T C, A Guide to Experiments in Quantum Optics, (Wiley-VCH, Weinheim), 2004.
16. Kimble H J, Dagenais M, Mandel L, Photon antibunching in resonance fluorescence, Phys Rev Lett, 39(1977)691; doi.org/10.1103/PhysRevLett.39.691.
17. Rarity J, Tapster P, Experimental violation of Bell’s inequality based on phase and momentum, Phys Rev Lett, 64 (1990)2495; doi.org/10.1103/PhysRevLett.64.2495
18. Pan J W, Bouwmeester D, Daniell M, Weinfurter H, Zeilinger A, Experimental test of quantum nonlocality in three- photon Greenberger–Horne–Zeilinger entanglement, Nature, 403(2000)515-519.
19. Avenhaus M, Laiho K, Chekhova M V, Silberhorn C, Accessing higher order correlations in quantum optical states by time multiplexing, Phys Rev Lett, 104(2010)063602; doi.org/10.1103/PhysRevLett.104.063602
20. Bouwmeester D, Pan J W, Mattle K, Eibl M, Weinfurter H, Zeilinger A, Experimental quantum teleportation, Nature, 390(1997)575-579.
21. Mattle K, Weinfurter H, Kwiat P G, Zeilinger A, Dense coding in experimental quantum communication, Phys Rev Lett, 76(1996)4656; doi.org/10.1103/PhysRevLett.76.4656
22. Lo H, Curty M, Tamaki K, Secure quantum key distribution, Nature Photonics, 8(2014)595-604.
23. Ma H X, Huang P, Bai D Y, Wang S Y, Bao W S, Zeng G H, Continuous-variable measurement-device-independent quantum key distribution with photon subtraction, Phys Rev A, 97(2018)042329; doi.org/10.1103/PhysRevA.97.042329
24. Jouguet P, Kunz-Jacques S, Leverrier A, Grangier P, Diamanti E, Experimental demonstration of long-distance continuous-variable quantum key distribution, Nature Photonics, 7(2013)378-381
25. Pirandola S, Ottaviani C, Spedalieri G, Weedbrook C, Braunstein S L, Lloyd S, Gehring T, Jacobsen C S, Andersen U L, High-rate measurement-device- independent quantum cryptography, Nature Photonics, 9(2015)397-402.
26. Pirandola S, Mancini S, Lloyd S, Braunstein S L, Continuous-variable quantum cryptography using two-way quan- tum communication, Nature Physics, 4(2008)726-730.
27. Liu W T, Sun S H, Liang L M, Yuan J M, Proof-of-principle experiment of a modified photon-number-splitting attack against quantum key distribution, Phys Rev A, 83(2011)042326; doi.org/10.1103/PhysRevA.83.042326
28. Zavatta A, Parigi V, Kim M, Bellini M, Subtracting photons from arbitrary light fields: experimental test of coherent state invariance by single-photon annihilation, New J Phys, 10(2008)123006; doi.org/10.1088/1367-2630/10/12/123006
29. Gerrits T, Glancy S, Clement T S, Calkins B, Lita A E, Miller A J, Migdall A L, Nam S W, Mirin R P, Knill E, Generation of optical coherent-state superpositions by number-resolved photon subtraction from the squeezed vacuum, Phys Rev A, 82(2010)031802; doi.org/10.1103/PhysRevA.82.031802
30. Malpani P, Alam N, Thapliyal K, Pathak A, Narayanan V, Banerjee S, Lower- and higher- order nonclassical properties of photon added and subtracted displaced Fock states, Ann Phys (Berlin), 531(2019)1800318; doi.org/10.1002/andp.201800318
31. Leoński W, Kowalewska-Kudłaszyk A, Quantum scissors–finite-dimensional states engineering, Progress in Optics, 56(2011)131-185.
32. Żukowski M, Zeilinger A, Horne M A, Realizable higher-dimensional two-particle entanglements via multiport beam splitters, Phys Rev A, 55(1997)2564; doi.org/10.1103/PhysRevA.55.2564
33. Agarwal G S, Quantum Optics, (Cambridge University Press, Cambridge), 2013.
34. Mewes M O, Andrews M, Kurn D, Durfee D, Townsend C, Ketterle W, Output coupler for Bose-Einstein condensed atoms, Phys Rev Lett, 78(1997)582; doi.org/10.1103/PhysRevLett.78.582
35. Torii Y, Suzuki Y, Kozuma M, Sugiura T, Kuga T, Deng L, Hagley E, Mach-zehnder Bragg interferometer for a Bose-Einstein condensate, Phys Rev A, 61(2000)041602; doi.org/10.1103/PhysRevA.61.041602
36. Thapliyal K, Banerjee S, Pathak A, Tomograms for open quantum systems: in (finite) dimensional optical and spin systems, Ann Phys, 366(2016)148-167.
37. Bennett C H, Brassard G, Quantum cryptography: public key distribution and coin tossing, International Conference on Computer System and Signal Processing, IEEE, 1984, (1984), pp 175-179.
38. Gisin N, Ribordy G, Tittel W, Zbinden H, Quantum cryptography, Rev Mod Phys, 74(2002)145; doi.org/10.1103/RevModPhys.74.145
39. Sharma V, Thapliyal K, Pathak A, Banerjee S, A comparative study of protocols for secure quantum communica- tion under noisy environment: single-qubit- based protocols versus entangled-state-based protocols, Quantum Inf Process, 15(2016)4681-4710.
40. Ghatak A, Pathak A, Sharma V P (editors), Light and its Many Wonders, (Viva Books, New Delhi), 2015.
41. Thapliyal K, Pathak A, Sen B, Pěrina J, Higher-order nonclassicalities in a codirectional nonlinear optical coupler: quantum entanglement, squeezing, and antibunching, Phys Rev A, 90(2014)013808; doi.org/10.1103/PhysRevA.90.013808
42. Thapliyal K, Samantray NL, Banerji J, Pathak A, Comparison of lower- and higher-order nonclassicality in photon added and subtracted squeezed coherent states, Phys Lett A, 381(2017)3178-3187.
43. Makhlin Y, Schön G, Shnirman A, Quantum-state engineering with Josephson-junction devices, Rev Mod Phys, 73(2001)357; doi.org/10.1103 /RevModPhys.73.357
44. Malpani P, Thapliyal K, Alam N, Pathak A, Narayanan V, Banerjee S, Quantum phase properties of photon added and subtracted displaced Fock states, Annalen der Physik, 531(2019)1900141; doi.org/10.1002/andp.201900141
45. Malpani P, Alam N, Thapliyal K, Pathak A, Narayanan V, Banerjee S, Manipulating nonclassicality via quantum state engineering processes: Vacuum filtration and single photon addition, Annalen der Physik (2019) In press.
46. Malpani P, Thapliyal K, Alam N, Pathak A, Narayanan V, Banerjee S, Impact of photon addition and subtraction on nonclassical and phase properties of a displaced Fock state, arXiv preprint arXiv:1908.04086 (2019).
47. Srikara S, Thapliyal K, Pathak A, Continuous variable B92 quantum key distribution protocol using single photon added and subtracted coherent states, arXiv preprint arXiv:1906.07768 (2019).
48. Weihs G, Zeilinger A, Photon statistics at beam-splitters: an essential tool in quantum information and teleportation, Coherence and Statistics of Photons and Atoms, (Wiley), 2001, pp 262–288.
49. Pathak A, Elements of Quantum Computation and Quantum Communication, (Taylor & Francis, New York), 2013.
50. Bennett C H, Brassard G, Crépeau C, Jozsa R, Peres A, Wootters W, Teleporting an unknown quantum state via dual classical and Einstein-Podolsky-Rosen channels, Phys Rev Lett, 70(1993)1895; doi.org/10.1103/PhysRevLett.70.1895
51. Shukla C, Thapliyal K, Pathak A, Hierarchical joint remote state preparation in noisy environment, Quantum Inf Process, 16(2017)205; https://doi.org/10.1007 /s11128-017-1654-3
52. Sisodia M, Shukla A, Thapliyal K, Pathak A, Design and experimental realization of an optimal scheme for tele- portation of an n-qubit quantum state, Quantum Inf Process, 16(2017)292 ; doi.org/10.1007/s11128-017-1744-2
53. Thapliyal K, Pathak A, Applications of quantum cryptographic switch: various tasks related to controlled quantum communication can be performed using Bell states and permutation of particles, Quantum Inf Process, 14(2015) 2599-2616.
54. Thapliyal K, Verma A, Pathak A, A general method for selecting quantum channel for bidirectional controlled state teleportation and other schemes of controlled quantum communication, Quantum Inf Process, 14(2015)4601-4614.
55. Bennett C H, Quantum cryptography using any two non orthogonal states, Phys Rev Lett, 68(1992)3121; doi.org/10.1103/PhysRevLett.68.3121
56. Bennett C H, Brassard G, Mermin ND, Quantum cryptography without Bell’s theorem, Phys Rev Lett, 68(1992) 557; doi.org/10.1103/PhysRevLett.68.557
57. Lo H, Curty M, Qi B, Measurement-device-independent quantum key distribution, Phys Rev Lett, 108(2012) 130503; doi.org/10.1103 /PhysRevLett.108.130503
58. Saxena A, Thapliyal K, Pathak A, Continuous variable controlled quantum dialogue and secure multiparty quantum computation, arXiv preprint arXiv:1902.00458 (2019).
59. Braunstein S L, Kimble H J, Teleportation of continuous quantum variables, Phys Rev Lett, 80(1998)869; doi.org/10.1103/PhysRevLett.80.869
60. Weedbrook C, Pirandola S, García-Patrón R, Cerf N J, Ralph T C, Shapiro J H, Lloyd S, Gaussian quantum information, Rev Mod Phys, 84(2012)621; doi.org/10.1103/RevModPhys.84.621
61. Mišta L(Jr), Filip R, Improving teleportation of continuous variables by local operations, Phys Rev A, 71(2005) 032342; doi.org/10.1103/PhysRevA.71.032342
62. Cerf N J, Levy M, Van Assche G, Quantum distribution of Gaussian keys using squeezed states, Phys Rev A, 63 (2001)052311; doi.org/10.1103 /PhysRevA.63.052311
63. Jacobsen C S, Madsen L S, Usenko V C, Filip R, Andersen U L, Complete elimination of information leakage in continuous-variable quantum communication channels, npj Quantum Inf, 4(2018)32; doi.org/10.1038/s41534-018-0084-0
64. Kok P, Munro W J, Nemoto K, Ralph T C, Dowling J P, Milburn G J, Linear optical quantum computing with photonic qubits, Rev Mod Phys, 79(2007)135; doi.org/10.1103/RevModPhys.79.135
65. O’brien J L, Optical quantum computing, Science, 318(2007)1567-1570.
66. Hong C, Ou Z Y, Mandel L, Measurement of subpicosecond time intervals between two photons by interference, Phys Rev Lett, 59(1987)2044; doi.org/10.1103/PhysRevLett.59.2044
67. Nielsen M A, Chuang I L, Quantum Computation and Quantum Information, (Cambridge University Press, New Delhi), 2010.
68. Ralph T C, Pryde G J, Optical quantum computation, Progress in Optics, 54(2010)209-269.
69. O’Brien J L, Pryde G J, White A G, Ralph T C, Branning D, Demonstration of an all-optical quantum controlled-NOT gate, Nature, 426(2003)264-271.
70. Knill E, Laflamme R, Milburn G, A scheme for efficient quantum computation with linear optics, Nature, 409 (2001)46-52.
71. Franson J D, Donegan M, Fitch M, Jacobs B, Pittman T, High-fidelity quantum logic operations using linear optical elements, Phys Rev Lett, 89(2002)137901; doi. 10.1103/PhysRevLett.89.137901
72. Ralph T C, Gilchrist A, Milburn G J, Munro W J, Glancy S, Quantum computation with optical coherent states, Phys Rev A, 68(2003)042319; doi.org/10.1103 /PhysRevA.68.042319
73. Gu M, Weedbrook C, Menicucci NC, Ralph TC, van Loock P, Quantum computing with continuous-variable clusters, Phys Rev A, 79(2009)062318; doi.org/10.1103/PhysRevA.79.062318
74. Tatham R, Korolkova N, Entanglement concentration with quantum nondemolition Hamiltonians, Phys Rev A, 89 (2014)012308; doi.org/10.1103 /PhysRevA.89.012308
75. Simon C, De Riedmatten H, Afzelius M, Sangouard N, Zbinden H, Gisin N, Quantum repeaters with photon pair sources and multimode memories, Phys Rev Lett, 98(2007)190503; /doi.org/10.1103/PhysRevLett.98.190503
76. Aspuru-Guzik A, Walther P, Photonic quantum simulators, Nature Physics, 8(2012)285-291.
77. Naikoo J, Thapliyal K, Banerjee S, Pathak A, Quantum Zeno effect and nonclassicality in a PT-symmetric system of coupled cavities, Phys Rev A, 99(2019)023820; doi.org/10.1103/PhysRevA.99.023820.
78. Sibson P, Erven C, Godfrey M, Miki S, Yamashita T, Fujiwara M, Sasaki M, Terai H, Tanner M G, Natarajan C M, Hadfield R H, O’Brien J L, Thompson M G, Chip-based quantum key distribution, Nat Commun, 8(2017)13984; doi: 10.1038/ncomms13984 (2017)
79. O’brien J L, Furusawa A, Vučkovic´ J, Photonic quantum technologies, Nature Photonics, 3(2009)687; doi.org/10.1038/nphoton.2009.229
80. Agarwal G S, Tewari S P, An all-optical realization of the quantum Zeno effect, Phys Lett A, 185(1994)139-142.
81. Noh T G, Counterfactual quantum cryptography, Phys Rev Lett, 103(2009)230501; doi.org/10.1103/PhysRevLett.103.230501
82. Brida G, Cavanna A, Degiovanni I P, Genovese M, Traina P, Experimental realization of counterfactual quantum cryptography, Laser Phys Lett, 9(2012)247-252.
83. Cao Y, Li YH, Cao Z, Yin J, Chen YA, Yin H L, Chen T Y, Ma X, Peng C Z, Pan J W, Direct counterfactual communication via quantum Zeno effect, Proc Nat Acad Sci, 114(2017)4920-4924.
84. Hosten O, Rakher M T, Barreiro J T, Peters N A, Kwiat P G, Counterfactual quantum computation through quantum interrogation, Nature, 439(2006)949-952.
85. Kong F, Ju C, Huang P, Wang P, Kong X, Shi F, Jiang L, Du J, Experimental realization of high-efficiency counter- factual computation, Phys Rev Lett, 115(2015)080501; doi.org/10.1103/PhysRevLett.115.080501
86. Elitzur A C, Vaidman L, Quantum mechanical interaction-free measurements, Found Phys, 23(1993)987-997.
87. Guo G C, Shi B S, Quantum cryptography based on interaction-free measurement, Phys Lett A, 256(1999)109-112.
88. Goldenberg L, Vaidman L, Quantum cryptography based on orthogonal states, Phys Rev Lett, 75(1995)1239; doi.org/10.1103/PhysRevLett.75.1239
89. Higgins B L, Berry D W, Bartlett S D, Wiseman H M, Pryde G J, Entanglement-free Heisenberg-limited phase estimation, Nature, 450(2007)393-396.
90. Kacprowicz M, Demkowicz-Dobrzański R, Wasilewski W, Banaszek K, Walmsley I, Experimental quantum- enhanced estimation of a lossy phase shift, Nature Photonics, 4(2010)357-360.
91. Giovannetti V, Lloyd S, Maccone L, Advances in quantum metrology, Nature Photonics, 5(2011)222-229.
92. Lanzagorta M, Quantum radar, Synthesis Lectures on Quantum Computing, 3(2011)1-139; doi.org/10.2200/S00384ED1V01Y201110QMC005)
93. Dhand I, D’Souza A, Narasimhachar V, Sinclair N, Wein S, Zarkeshian P, Poostindouz A, Simon C, Understand- ing quantum physics through simple experiments: from wave-particle duality to Bell’s theorem, arXiv preprint arXiv:1806.09958 (2018).
94. Polino E, Agresti I, Poderini D, Carvacho G, Milani G, Lemos GB, Chaves R, Sciarrino F, Device-independent test of a delayed choice experiment, Phys Rev A, 100 (2019)022111; doi.org/10.1103/PhysRevA.100.022111
95. Irvine WT, Hodelin JF, Simon C, Bouwmeester D, Realization of Hardy’s thought experiment with photons, Phys Rev Lett, 95(2005)030401; doi.org/10.1103 /PhysRevLett.95.030401.
96. Grangier P, Roger G, Aspect A, Experimental evidence for a photon anticorrelation effect on a beam splitter: a new light on single-photon interferences, Europhys Lett, 1(1986)173-179.
97. Li Z Y, Weak-measurement Mach-Zehnder interferometer for testing wave-particle duality, Europhys Lett, 117 (2017)50005; doi.org/10.1209/0295-5075/117/50005
98. Gisin N, Pironio S, Sangouard N, Proposal for implementing device-independent quantum key distribution based on a heralded qubit amplifier, Phys Rev Lett, 105(2010)070501; doi.org/10.1103/PhysRevLett.105.070501
99. Aspelmeyer M, Kippenberg T J, Marquardt F, Cavity optomechanics, Rev Mod Phys, 86(2014)1391; doi.org/10.1103/RevModPhys.86.1391
100. Heeres R.W, Kouwenhoven LP, Zwiller V, Quantum interference in plasmonic circuits, Nature Nanotechnology, 8(2013)719-722.
101. Giri S, Thapliyal K, Sen B, Pathak A, Nonclassicality in an atom–molecule Bose–Einstein condensate: Higher-order squeezing, antibunching and entanglement, Physica A, 466(2017)140-152.
102. Thapliyal K, Pathak A, Sen B, Pĕrina J, Nonclassical properties of a contradirectional nonlinear optical coupler, Phys Lett A, 378(2014)3431-3440.
103. Thapliyal K, Pathak A, Pĕrina J, Linear and nonlinear quantum Zeno and anti-Zeno effects in a nonlinear optical coupler, Phys Rev A, 93(2016)022107; doi.org/10.1103/PhysRevA.93.022107
104. Mukhopadhyay A, Sen B, Thapliyal K, Mandal S, Pathak A, Interaction of light and semiconductor can gener- ate quantum states required for solid-state quantum computing: entangled, steered and other nonclassical states, Quantum Inf Process, 18(2019)234; doi.org/10.1007/s11128-019-2344-0
105. Xiao Y, Klein M, Hohensee M, Jiang L, Phillips D F, Lukin M D, Walsworth R L, Slow light beam splitter, Phys Rev Lett, 101(2008)043601; doi.org/10.1103 /PhysRevLett.101.043601
106. Hénault F, Quantum physics and the beam splitter mystery, The Nature of Light: What are Photons? VI, 9570 (2015) 95700Q, International Society for Optics and Photonics ; doi.org/10.1117/12.2186291.
107. Holbrow C, Galvez E, Parks M, Photon quantum mechanics and beam splitters, Am J Phys, 70(2002)260-265.
108. Zeilinger A, General properties of lossless beam splitters in interferometry, Am J Phys, 49(1981)882-883.